- Creating liquid biofuels from human waste shows promise as a way to meet one of alternative energy’s greatest challenges: reducing the transportation sector’s heavy carbon footprint. The good news is there is a steady supply stream where waste is treated.
- Humanity produces millions of tons of sewage sludge annually via wastewater treatment. Existing disposal methods include landfilling, application on agricultural land, and incineration; each with social and environmental consequences.
- Harnessing the carbon-rich potential of sludge as a transportation fuel for planes, ships and trucks is part of a drive toward zero waste and creating a circular economy, say experts. A host of projects are underway to prove the effectiveness of various methods of turning all this crud into biocrude.
- Some techniques show promise in lab and pilot tests, but large-scale industrial plants have yet to be built. Using pollutant-laden sewage sludge as a biofuel comes with its own environmental concerns, but lacking a silver-bullet solution to the human waste problem, it could be part of a suite of best alternatives.
Last year, a car fueled by human waste toured the European countryside, covering more than 2,000 kilometers (1,200 miles). It was the culmination of To-Syn-Fuel, a pathfinding project using technology developed by Germany’s Fraunhofer Institute UMSICHT to make 50,000 liters (13,200 gallons) of biocrude oil from 500 tons of sewage sludge.
The trip marked a milestone in global efforts to convert human waste into a viable transportation fuel, according to Robert Daschner, head of the department of renewable energy at Fraunhofer UMSICHT, which led the multi-partner project funded by the EU’s Horizon 2020 program. Using To-Syn-Fuel can achieve carbon savings of up to 85% compared to fossil fuels, he wrote to Mongabay in an email.
But that historic EU road trip was only an intermediary goal: Next up is the construction of a larger-scale crud-to-crude facility in Germany, built upon lessons learned from a smaller proof-of-concept demonstration plant. The aim is to process up to 400,000 metric tons of sewage sludge into “sustainable aviation fuel” by 2030, Daschner wrote.
If this, or other promising crud-to-crude pilot projects, achieve truly scaled-up success, science and industry could crack one of the hardest nuts for realizing a post-fossil fuel economy: a transportation liquid fuel, with low-carbon potential to power cars, trucks, jets and ships.
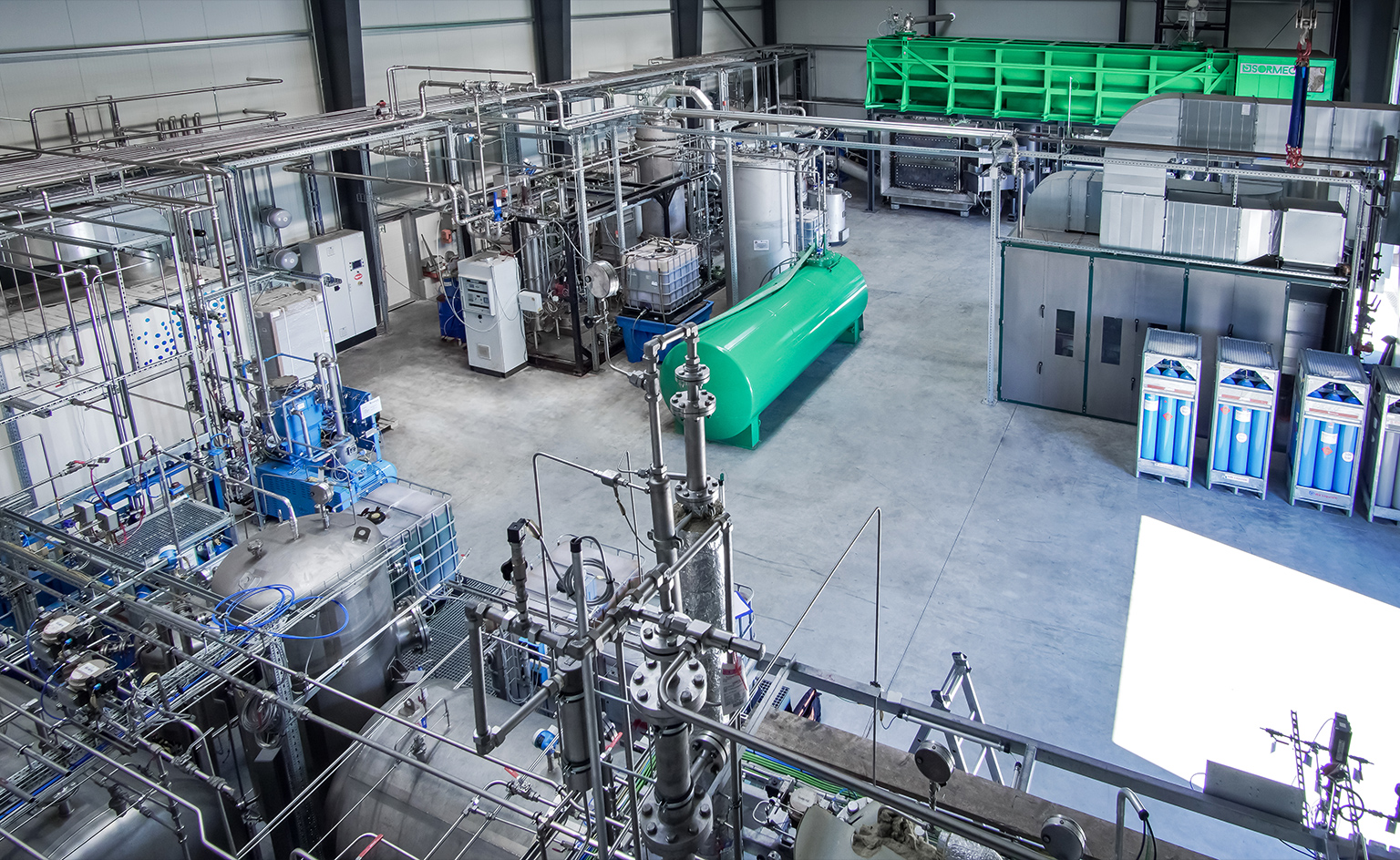
The case for human waste
Sewage sludge is a carbon- and nutrient-rich mud-like residue left behind after wastewater treatment. Transportation experts don’t see this leftover as waste, but instead as a form of refinable gold: as renewable feedstock from which to make liquid biofuel.
Some experts even say that harnessing the power potential of wastewater and sewage sludge as part of a circular economy may be vital to beating climate change. But achieving that goal, while offering big opportunities, also presents big challenges.
Millions of tons of sewage sludge are created annually at wastewater treatment plants, though much of it now ends up in landfills or is incinerated. Treated sludge, known as “biosolids,” is also composted and can be spread as an agricultural amendment.
In 2021, the U.S. produced 4.5 million metric tons of sludge, according to the Environmental Protection Agency. Of that, nearly 2 million metric tons were applied to land; 633,000 metric tons were incinerated, and 1.9 million metric tons were landfilled. While the European Union produces less sewage sludge, around 10 million metric tons yearly, its use follows a broadly similar pattern.
Each sewage sludge disposal route causes its own major environmental problems. Spreading sludge on farmland, for example, can release pollutants into soils, contaminating vast areas and even poisoning people with toxic heavy metals, PFAS “forever chemicals,” and microplastics. This problem led to a ban on sewage sludge spreading in the U.S. state of Maine last year.
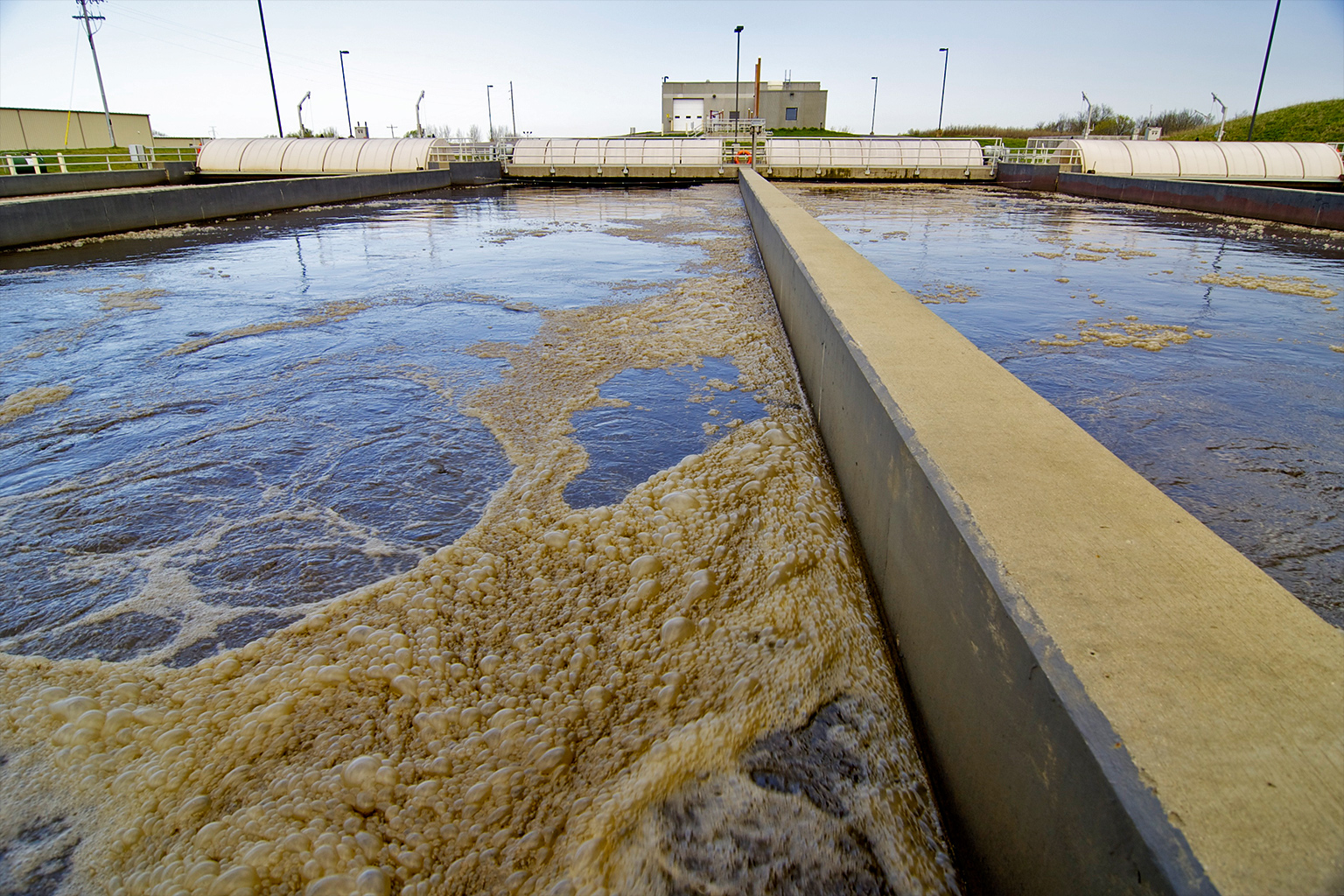
Wastewater treatment itself is a carbon-intensive process, accounting for as much as 5% of humanity’s greenhouse gas emissions, according to some estimates. Currently just 20% of global wastewater is treated, meaning that as waste treatment service expands, its environmental footprint could grow with it.
“Wastewater treatment plant operators often have to burn the sludge, and this creates huge greenhouse gas emissions,” Frédéric Pitre, a professor at the University of Montreal in Canada, told Mongabay in an interview. “It’s a challenge to find ways to give value to this sludge.”
Generating energy (in the form of biogas) and recovering nutrients (such as nitrogen and phosphorous, useful as fertilizers) are two other alternatives for extracting that “value,” with waste treatment operators already creating power from poop to shrink their carbon footprint, says Lillian Zaremba, collaborative innovations program manager for Metro Vancouver’s liquid waste service. Using a process known as anaerobic digestion, sewage sludge is sometimes used as feedstock to produce biogas in the form of methane, which is typically pumped back into treatment facilities to meet their energy generation needs.
“We use the biomethane that comes out of anaerobic digestion in the [waste treatment] plants, and then whatever we can’t use, we clean up for sale,” Zaremba explains. “It’s displacing fossil fuel gas [use].”

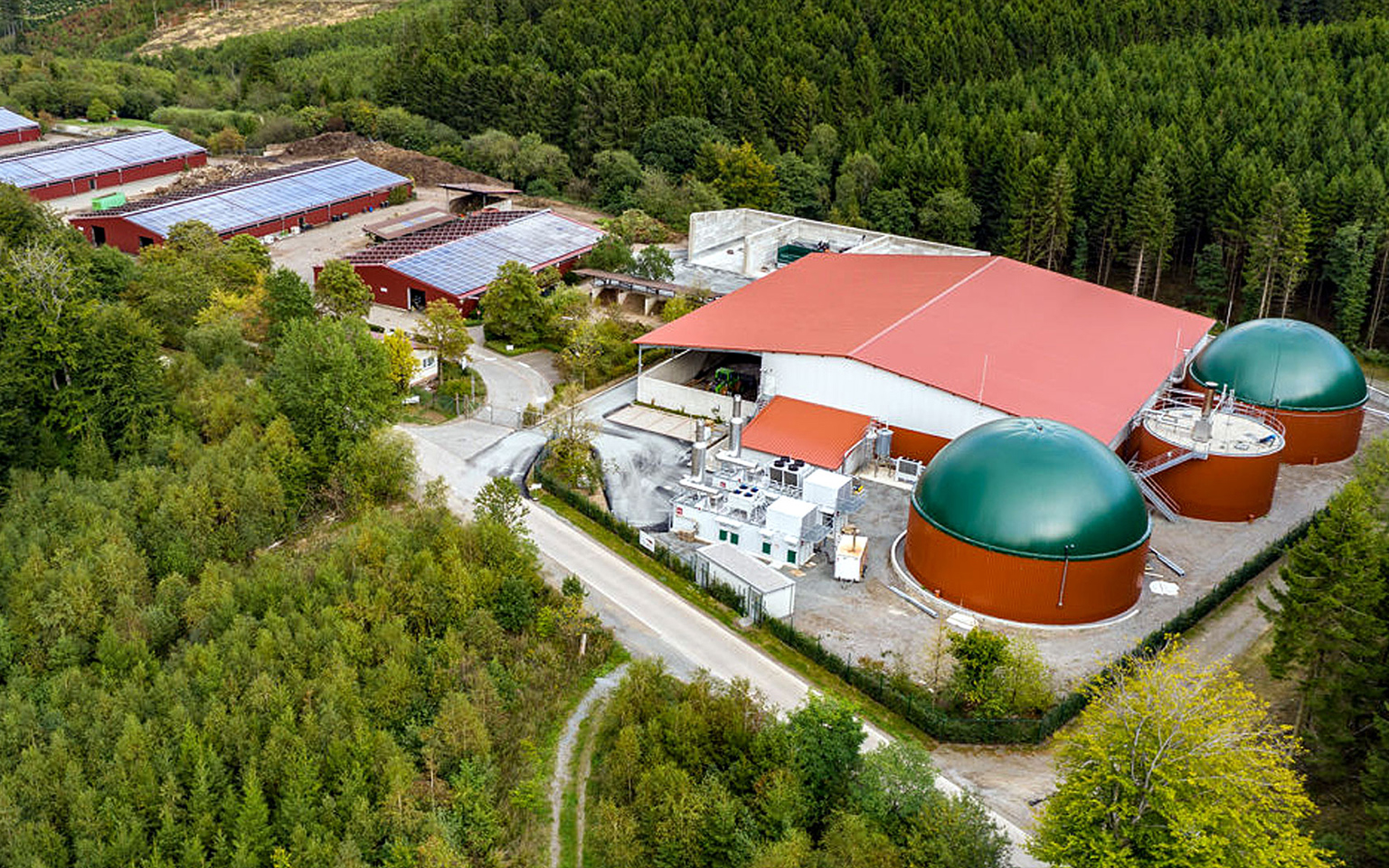
From human waste to liquid biofuel
A host of technologies are under investigation to turn sewage sludge into commercially viable liquid biofuels to meet transportation needs. A review paper published last year outlines options including “biological processes, thermochemical technologies, bioelectrochemical processing, biorefineries and others.”
Though small-scale demonstrations of liquid biofuel generation from sludge have seen success, no large-scale industrial facilities are operational yet. “[A]lthough tested and validated at a laboratory or pilot scale, [the various processing techniques] still remain in their infancy from the industrial application aspect,” says the review paper.
Proponents tout the advantages of sludge-based biofuels, noting that they can avoid many of the common criticisms of other liquid biofuel feedstocks, including corn, palm oil or vegetable oil. Sludge as a feedstock offers zero competition with food or agricultural land use, and land conversion is avoided — issues that can drive down the sustainability and up the carbon intensity of various biofuels.
Laureano Jiménez, a chemical engineer at Spain’s University of Rovira i Virgili, and his team conducted a sustainability analysis of different liquid biofuel feedstocks; waste sources, such as sewage sludge, came out tops. “We are not saying creating biofuels from sewage is the best option, but it’s one of them,” he emphasized.
“We are using waste that in any case will be burned or landfilled,” Salvador Ordoñez at the Department of Chemical and Environmental Engineering at the University of Oviedo, Spain, added. That can make sludge-based biofuel more sustainable, he believes.
Transforming crud to crude
Hydrothermal liquefaction (HTL), using pressure and heat, is one route for turning municipal waste into biocrude, and it’s considered one of the options with the greatest potential to create fuels for shipping and possibly sustainable aviation fuels. Last year, researchers working on a pilot-scale project at Denmark’s Aarhus University announced a “breakthrough” as biocrude was successfully produced using feedstocks including sewage sludge.
In Canada, an HTL demonstration project under development by Metro Vancouver is planned to go operational by 2025, turning around 10 metric tons of wet sewage sludge into five barrels of biocrude daily. Though that’s a drop in the bucket compared to global fuel needs, starting small is a crucial proof of concept before scaling up further, Zaremba said. Similar pilot projects are underway elsewhere.
Stian Hegdahl, a Ph.D. candidate at Norway’s University of Bergen, also believes HTL shows promise for turning human waste into biofuel. His lab-scale research uses sludge that has already gone through the anaerobic digestion process.
He cautions that biofuel production isn’t without its environmental problems. The conversion of sludge to fuel produces both solid and liquid waste, for which disposal would be required, and you “would have to consider how this is done [safely],” he said.
Another hurdle: Crud-to-crude refineries, if they’re to meet large-scale demand, may need to truck in sludge from numerous waste treatment plants, depending on their geographic location. Hegdahl carried out “very rough” calculations on the amount of biocrude his hometown of Bergen could make, and found that the total waste of its 300,000 inhabitants could only produce “thousands of tons” of fuel — a figure dwarfed by current fossil fuel output. This could bump up costs due to logistics, making it economically unviable.
“You need to collect [sewage sludge] from several places into one [biofuel] plant to be able to produce enough for anybody to actually care about it,” he explained.
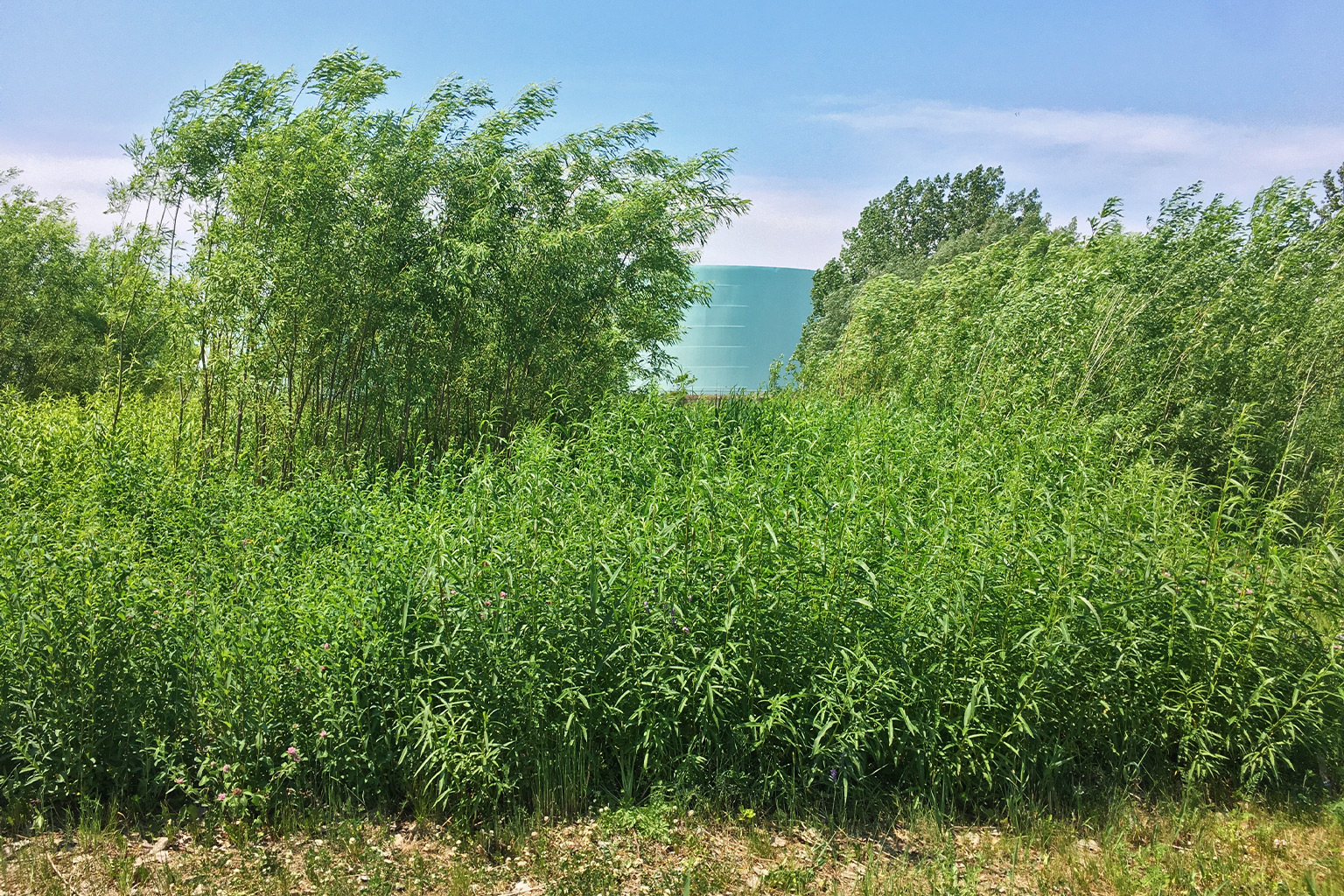
A Canadian project took an alternative approach to HTL, testing instead the capacity of fast-growing willow to treat wastewater as a nature-based solution; a purpose for which it proved effective. Researchers then found that this waste treatment solution could also provide a sustainable source of biofuels. Treating wastewater with willow greatly increased the plant’s biomass, explains Pitre, who was part of the research team. That biomass could then be made into liquid fuel, or other products such as bioplastics, an outcome he describes as a win-win solution.
“We’re not using farmland; we are not using food, and we are treating an environmental problem,” he said. Willow is just one of several plant species that could be used for such purposes, according to research. “We are either treating wastewater at a cost that is much lower than traditional wastewater treatment plants, or we are extracting contaminants from the soil and producing biomass that would otherwise be unused.”
No matter whether the feedstock is sewage sludge, willow or other organic material, the regulation of biofuel production poses yet another implementation hurdle. Any “demonstration [project] would need to be accepted by local jurisdiction and local government as a suitable option for the treatment of wastewater,” before going mainstream, Pitre noted.
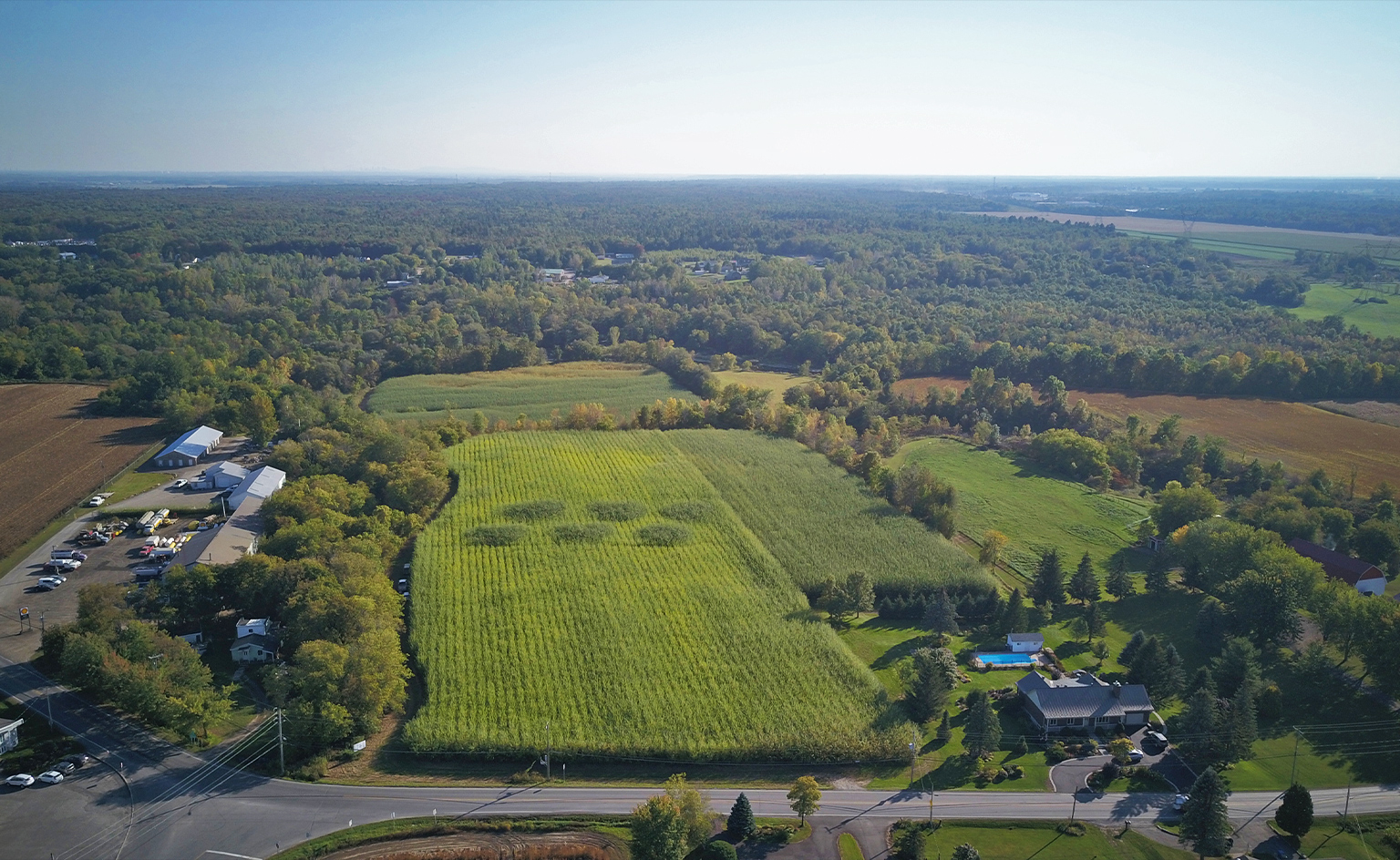

The question of carbon intensity
Even as entrepreneurs work to turn lab- and pilot-scale demonstrations into industrial-scale projects, analysts question just how much carbon savings these various biofuels offer.
In some cases, the touted savings linked to these new “low-carbon” fuels remain to be confirmed. Their efficiency perhaps depends most on where the line is drawn in accounting, argues Zaremba.
“We’re seeing that the carbon intensity of the biocrude is 90% lower than fossil crude,” she said. But that high figure is because benefits are being calculated after the sewage has “already been collected and treated.” Which means that none of the “additional carbon emissions from that part of the process” are counted in the 90% reduction.
Likewise, creating fuel using the HTL process requires hydrogen, and making hydrogen has its own carbon footprint. Producing “green hydrogen” from renewable sources is one way to unlock carbon savings, but currently the overwhelming majority of hydrogen is made using fossil fuels; in 2021, renewable energy produced just 1% of hydrogen globally.
“This is something that we are on our way to solve, but it’s still far away,” Carlos Pozo, a lecturer at the University of Rovira I Virgili, said. “Most of current hydrogen production is not green by any means, so this will be another environmental challenge.”
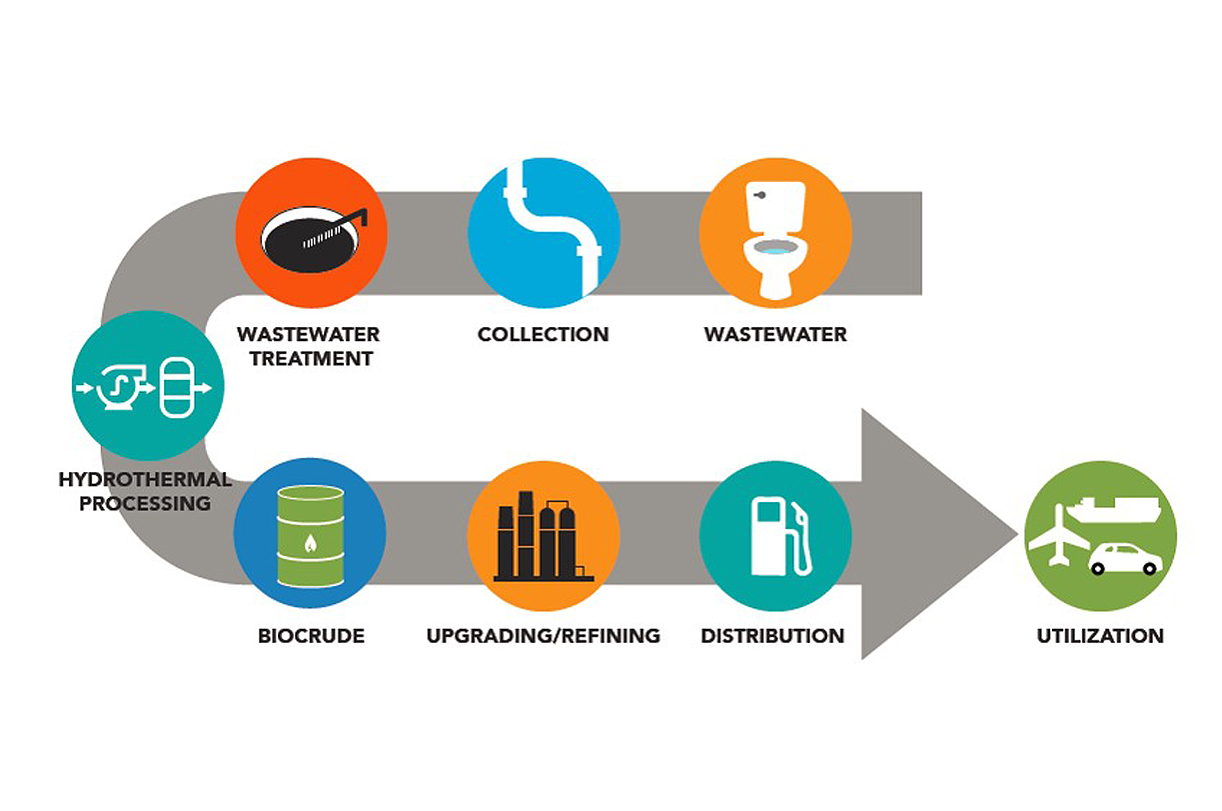
In To-Syn-Fuel’s case, carbon savings could be enhanced by sequestering solid carbon waste in the form of biochar created during the sludge-to-biofuel conversion process, Daschner wrote. But, he adds, a full carbon accounting has yet to be assessed: “As this is a research and demonstration plant, only limited statements can be made about the life-cycle assessment of a full-scale production plant.” But he notes that evaluating “the feedstock and eventual feedstock pre-treatment steps” will be key to determining carbon savings.
Another conundrum: the toxic contaminants found in wastewater. Sludge isn’t only packed with extractable carbon and agricultural nutrients, it also comes laden with undesirable pollutants. Depending on the method used to make biofuels, these contaminants remain a headache, and may need to be landfilled or burned.
“In this sense, we are not removing [pollutants] from the environment,” Jiménez said. “There are no easy solutions.”
Zaremba recognizes the contaminant problem, but she reports that HTL is “very promising in terms of destroying compounds of environmental concern.” Research is ongoing to understand what happens to pollutants during the biofuel conversion process, she said. “This pilot project is a great opportunity for [learning] that.”
A paper published last year found that some PFAS forever chemicals are not completely eliminated during HTL conversion, suggesting that “other treatment approaches for PFAS removal from sewage sludge need to be identified.”
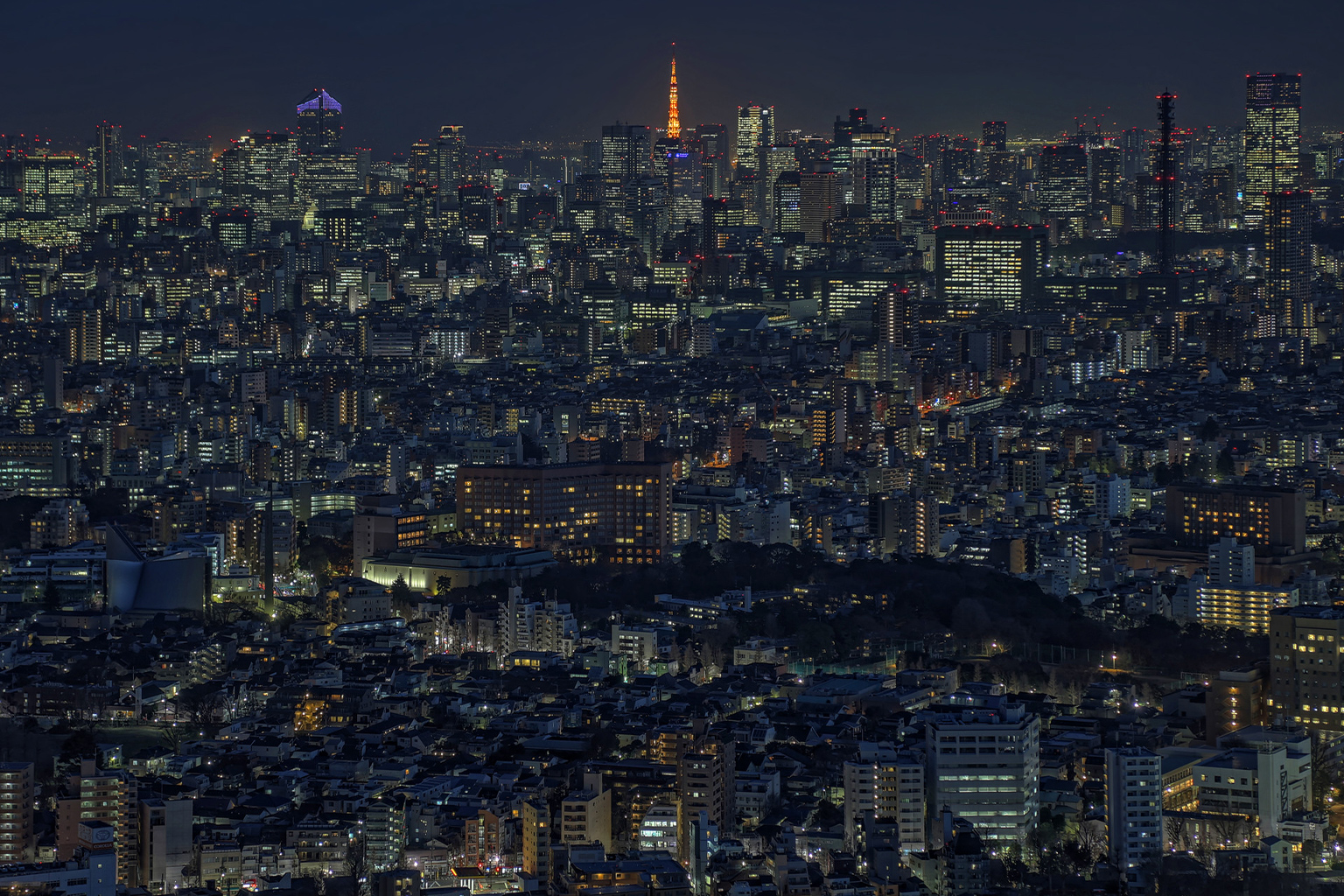
Aside from the environmental and technological difficulties, experts point to economic and political hurdles to taking sludge-based biofuels mainstream. For example, convincing municipal wastewater treatment plant operators, and taxpayers, to add potentially costly technology to plants to facilitate the manufacture of biofuels may be a difficult sell, says Ordoñez.
“When you try to develop a new technology, the market is important,” he noted. “We train as scientists to look at [solving] the technological part [of the problem], but the market is also important.”
A multitude of crud-to-crude options are in development today, but whether any will break through and produce sufficient fuel to significantly reduce transportation carbon emissions remains to be seen.
It does seem likely that crud-to-crude could become one of a suite of solutions addressing swelling humanity’s 21st-century waste disposal problem — solutions that now include using sewage sludge to make biogas, fertilizer and soil amendments, or disposal via incineration or depositing in landfills.
“We have to find a proper treatment in order to incorporate [sewage sludge] into a circular economy,” Jiménez said. Producing “biofuels is one of those alternatives.”
Hopes hinge on the outcomes of various efforts to scale up: “We’ve had a lot of interest from our peers across North America and we’ve had folks in Europe reach out to us,” says Zaremba. “Everyone is waiting for somebody to go first, so they can then make the case for implementing it themselves … that pilot scale — [that] intermediate step [which] will allow us to build a really compelling [biofuel] business case.”
Banner image: During their five-year project, To-Syn-Fuel converted more than 500 metric tons of dried sewage sludge into 50,000 liters (13,200 gallons) of oil. The same process can be a source of “green” hydrogen, the project team says. To-Syn-Fuel was funded by the EU and included a range of academic, research, and industry partners. Image © Fraunhofer UMSICHT.
Innovative sewage solutions: Tackling the global human waste problem
Citations:
Domini, M., Bertanza, G., Vahidzadeh, R., & Pedrazzani, R. (2022). Sewage sludge quality and management for circular economy opportunities in Lombardy. Applied Sciences, 12(20), 10391. doi:10.3390/app122010391
Rolsky, C., Kelkar, V., Driver, E., & Halden, R. U. (2020). Municipal sewage sludge as a source of microplastics in the environment. Current Opinion in Environmental Science & Health, 14, 16-22. doi:10.1016/j.coesh.2019.12.001
Demirbas, A., Taylan, O., & Kaya, D. (2016). Biogas production from municipal sewage sludge (MSS). Energy Sources, Part A: Recovery, Utilization, and Environmental Effects, 38(20), 3027-3033. doi:10.1080/15567036.2015.1124944
Cecconet, D., & Capodaglio, A. G. (2022). Sewage sludge biorefinery for circular economy. Sustainability, 14(22), 14841. doi:10.3390/su142214841
Cabrera-Jiménez, R., Mateo-Sanz, J. M., Gavaldà, J., Jiménez, L., & Pozo, C. (2022). Comparing biofuels through the lens of sustainability: A data envelopment analysis approach. Applied Energy, 307, 118201. doi:10.1016/j.apenergy.2021.118201
Chisti, Y. (2019). Introduction to algal fuels. In Biofuels from algae (pp. 1-31). Elsevier. doi:10.1016/B978-0-444-64192-2.00001-9
Fan, Y., Hornung, U., & Dahmen, N. (2022). Hydrothermal liquefaction of sewage sludge for biofuel application: A review on fundamentals, current challenges and strategies. Biomass and Bioenergy, 165, 106570. doi:10.1016/j.biombioe.2022.106570
Lozano, E. M., Løkke, S., Rosendahl, L. A., & Pedersen, T. H. (2022). Production of marine biofuels from hydrothermal liquefaction of sewage sludge. Preliminary techno-economic analysis and life-cycle GHG emissions assessment of Dutch case study. Energy Conversion and Management: X, 14, 100178. doi:10.1016/j.ecmx.2022.100178
Bashir, M. A., Lima, S., Jahangiri, H., Majewski, A. J., Hofmann, M., Hornung, A., & Ouadi, M. (2022). A step change towards sustainable aviation fuel from sewage sludge. Journal of Analytical and Applied Pyrolysis, 163, 105498. doi:10.1016/j.jaap.2022.105498
Hegdahl, S. H., Ghoreishi, S., Løhre, C., & Barth, T. (2023). Exploring hydrothermal liquefaction (HTL) of digested sewage sludge (DSS) at laboratory pilot scale using experimental design. Research Square. doi:10.21203/rs.3.rs-2516400/v1
Sas, E., Hennequin, L. M., Frémont, A., Jerbi, A., Legault, N., Lamontagne, J., … Pitre, F. E. (2021). Biorefinery potential of sustainable municipal wastewater treatment using fast-growing willow. Science of The Total Environment, 792, 148146. doi:10.1016/j.scitotenv.2021.148146
Rodriguez-Dominguez, M. A., Biller, P., Carvalho, P. N., Brix, H., & Arias, C. A. (2021). Potential use of plant biomass from treatment wetland systems for producing biofuels through a biocrude green-biorefining platform. Energies, 14(23), 8157. doi:10.3390/en14238157
Zhang, W., & Liang, Y. (2022). Hydrothermal liquefaction of sewage sludge — Effect of four reagents on relevant parameters related to biocrude and PFAS. Journal of Environmental Chemical Engineering, 10(1), 107092. doi:10.1016/j.jece.2021.107092
FEEDBACK: Use this form to send a message to the author of this post. If you want to post a public comment, you can do that at the bottom of the page.