- Pacific salmon can play a key role in transporting nutrients from marine to freshwater and terrestrial ecosystems.
- In the past, Pacific salmon and other anadromous fish that spawn in freshwater and spend part of their life in the ocean likely played a much larger role in global nutrient cycles, scientists find.
- But today, many populations of Pacific salmon and other anadromous fish are under pressure from habitat loss, overfishing, climate change, dams and other pressures that have greatly reduced their numbers, weight and ability to migrate freely.
- Population declines could further curtail their role in global nutrient transport in future, with increasing consequences, especially for nutrient-poor ecosystems that have relied in the past on migratory fish for significant nutrient additions.
Yukon River chinook salmon boast the longest freshwater migration in North America, traveling more than 3,000 kilometers (nearly 2,000 miles) from their spawning grounds in Canada to the mouth of the Bering Sea in Alaska. A smolt preparing to enter the ocean is small enough to lay across the palm of your hand. But by the time it returns four or five years later to swim back upriver, it will be about a meter long (more than 3 feet), and weigh 14 kilograms (more than 30 pounds).
Not all Pacific salmon (Oncorhynchus spp.) travel as far, or grow as big, as the Yukon River chinook (O. tshawytscha), but they all make the journey from freshwater spawning grounds to the ocean, then return to their natal streams to spawn and then die en masse. Those remarkable journeys connect and nurture distant ecosystems.
That’s because salmon gain most of their body mass in the ocean, and when spawning adults travel back inland to die, they ferry energy and nutrients from marine to terrestrial ecosystems. Those migrating salmon are eaten by bears, eagles and a slew of other predators. Scavengers devour carcasses left rotting in streambeds, or discarded in the forest along streams by other carnivores. Ultimately the nutrients diffuse throughout the ecosystem. The young return to the sea to reinvigorate the cycle, in a dance that has lasted unknown centuries — until modern humans began interfering.
Today, researchers can trace the pathway of these nutrients using the “salmon signature,” an isotope of marine nitrogen associated with the migrating fish. John Reynolds, a professor of aquatic ecology and conservation at Canada’s Simon Fraser University, has been searching for that salmon signature in the temperate rainforest of British Columbia’s central coast for more than 15 years.
His lab has published numerous studies showing that nutrient additions from salmon influence wildflower development; the productivity of salmon berry bushes; the territory size of Pacific wrens (Troglodytes pacificus); the density of salmon and other freshwater fish; and more. The salmon signature can even be seen from space: on satellite imagery, the greenness of riparian forests is higher following years of high salmon abundance.

Finding an echo of great migrations past
As diverse as these many impacts are today, they’re only detectable within 100 m (330 ft) or so of streams, Reynolds says. But at one time, before widespread extinctions and population declines, animals, including salmon, may have been much more important in global nutrient cycles.
That’s according to a 2016 study published in the Proceeding of the National Academy of Science. In prehistoric times, when woolly mammoths and other large megafauna roamed the Earth, researchers found that animals transported 94% more nutrients than today, simply by feeding in one place and defecating and dying in another.
The 2016 study looked not only at that remote past, but at how more recent declines in whales, fish, seabirds and other species have changed and diminished nutrient cycling. Dramatic declines in species of anadromous fish and. to a lesser extent, seabirds, mean their capacity to move nutrients — specifically phosphorus — from sea to land has declined by 96%.
“What we tried to do in that paper was take a historical perspective, not a current perspective where the animals are very limited in motion, [and] the populations tend to be quite low,” says study lead author Christopher Doughty, associate professor of ecoinformatics at Northern Arizona University. “Salmon are a great example where we’ve put up a ton of dams [and] their population numbers are vastly lower than what they were previously.”
While the study modeled only the transport of phosphorus, the results are broadly applicable to other nutrients as well, Doughty says. And though the figures are rough, they are, if anything, an underestimate, he adds. The analysis only looked at declines in population size, and didn’t take into account the barriers that stop animals moving long distances, or the linkages between different species.
Humans have disrupted global nutrient cycles in other ways. Our extensive use of synthetic fertilizers, for example, has resulted in the dumping of massive quantities of nitrogen and phosphorus into the environment; more in some places, less in others. This creates a gross imbalance in natural systems, particularly streams and estuaries, where overfertilization can lead to a flush of algae growth resulting in waterway anoxia and massive fish die-offs.
Scientists warn that by doing so we’ve destabilized the natural cycling of these life-giving nutrients to such a high degree that we’ve turned them toxic, and have now transgressed the planetary boundary for nitrogen and phosphorous biogeochemical flows, according to a 2023 Science Advances paper, putting a key Earth operating system vital for life at risk.
“We’re in a weird system, right now. There’re places [where] we have way too many nutrients, but then there’re also places that have way too few,” says Doughty. “Broadly speaking, animals can play a key role in reducing these extreme concentration gradients that we have these days.” But only if those animals exist in sufficient numbers, and are able to move freely.


The case of Pacific salmon
While a number of species shift nutrients from sea to land, there’s been far more research done on nutrient cycling by Pacific salmon than on other species of anadromous fish. That’s partly because Pacific salmon may play an exceptionally large role in the process, due to their life history. Importantly, they die after spawning, so with each migration all their biomass is transferred to freshwater streams and land. Also, they are fairly large bodied, and so contain high levels of nutrients. Adding to that, the habitats in which they spawn typically have low levels of nutrients, so their contribution may have a bigger effect.
Likewise, the modern pressures Pacific salmon face are common to many anadromous fish (and indeed other species). Many of the salmon’s long-established migratory routes have been blocked or partially obstructed by hydroelectric dams, culverts or other obstacles. Crucial habitat has been degraded by logging, urbanization or resource extraction. Freshwater habitats are polluted: For example, in 2020 researchers discovered that an ongoing mass die-off of coho salmon (O. kisutch) in the U.S. was caused by a chemical additive found in car tires, washed from roads into streams. Many populations are also overfished, and there have been declines in genetic diversity partially because of the proliferation of hatchery-bred fish.
Those pressures mount up. “We’re asking a lot of salmon these days, in terms of coping with all these different pressures that are happening in the watershed, and then [comes] this massive game changer of climate change,” says Jonathon Moore, a professor of aquatic ecology and conservation at Simon Fraser University.
Climate change stresses many species worldwide, but it brings some special challenges for salmon and other anadromous fish because they have to contend with changes in each of the vastly different ecosystems they pass through.
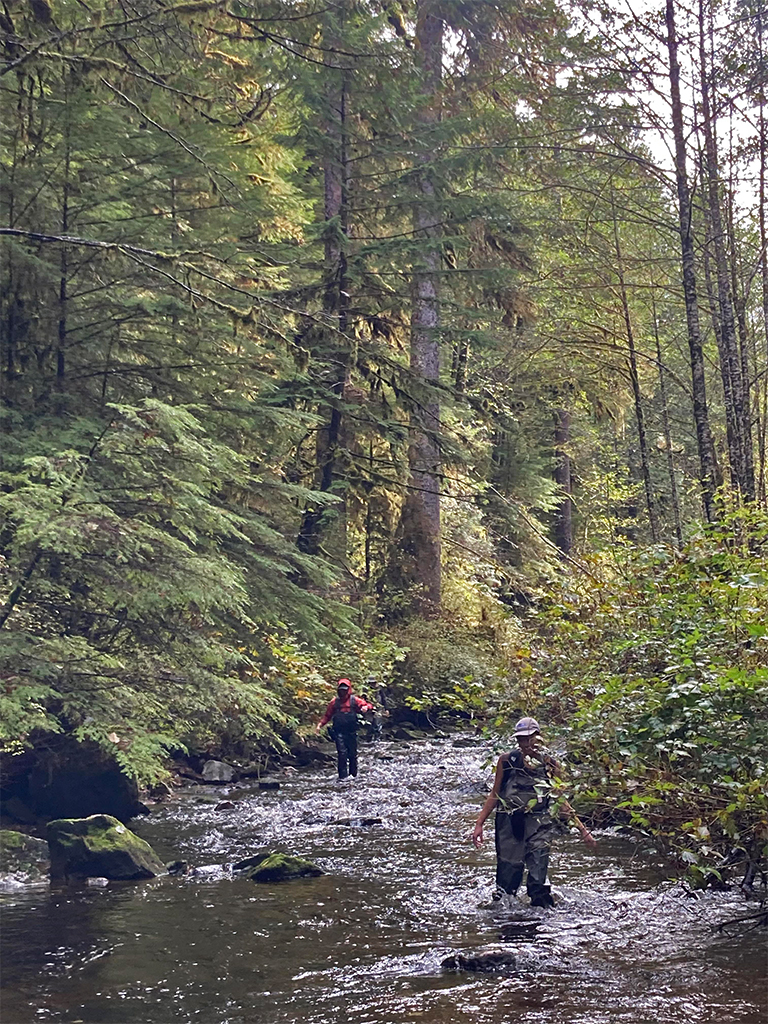
For Pacific salmon, a critical issue arises because they are adapted to live in cool water; at water temperatures warmer than 17° Celsius (63° Fahrenheit) they become physiologically stressed, while temperatures above 23°C (73°F) can be lethal with prolonged exposure.
As the Earth heats up, once-cool freshwater streams and rivers get warmer due to hotter summers, lower snowpacks and receding headwater glaciers. Drought is also becoming more common; it can prevent adults from reaching spawning grounds in freshwater, or strand juveniles in isolated pools.
In the salmon’s ocean habitat, warmer waters favor smaller, less nutritious types of zooplankton, an important food source. At the same time, warmer water causes their metabolism to speed up, so that they need more food. Marine heat waves, such as the one lasting from 2014-16 known as “the blob,” can lead to high mortality for salmon and other fish. That means fewer adults returning to freshwater to spawn.
Climate change is also a particularly thorny problem for migratory species because it can mess with the timing of their migrations. Recent research shows that this could be a problem for Pacific salmon too. Overall, juveniles are migrating to the ocean earlier in the year, according to a May 2023 study published in Nature Ecology & Evolution, with the young fish taking their movement cues from changing freshwater conditions. But ocean conditions are changing at a different rate. That means the young fish are arriving in estuaries at the wrong time. And because the first few months in the ocean are critical for a young salmon’s survival into adulthood, a mismatch between migration timing and estuarine food availability could have large impacts.
“Right now there isn’t a huge mismatch, but it could get worse” as climate change escalates, says study author Samantha Wilson, a research scientist at Simon Fraser University.
As a result of all these pressures, many, though not all, Pacific salmon populations in North America are declining. About half of British Columbia Pacific salmon populations are falling, and 28 populations in the U.S. Pacific Northwest are listed under the Endangered Species Act.
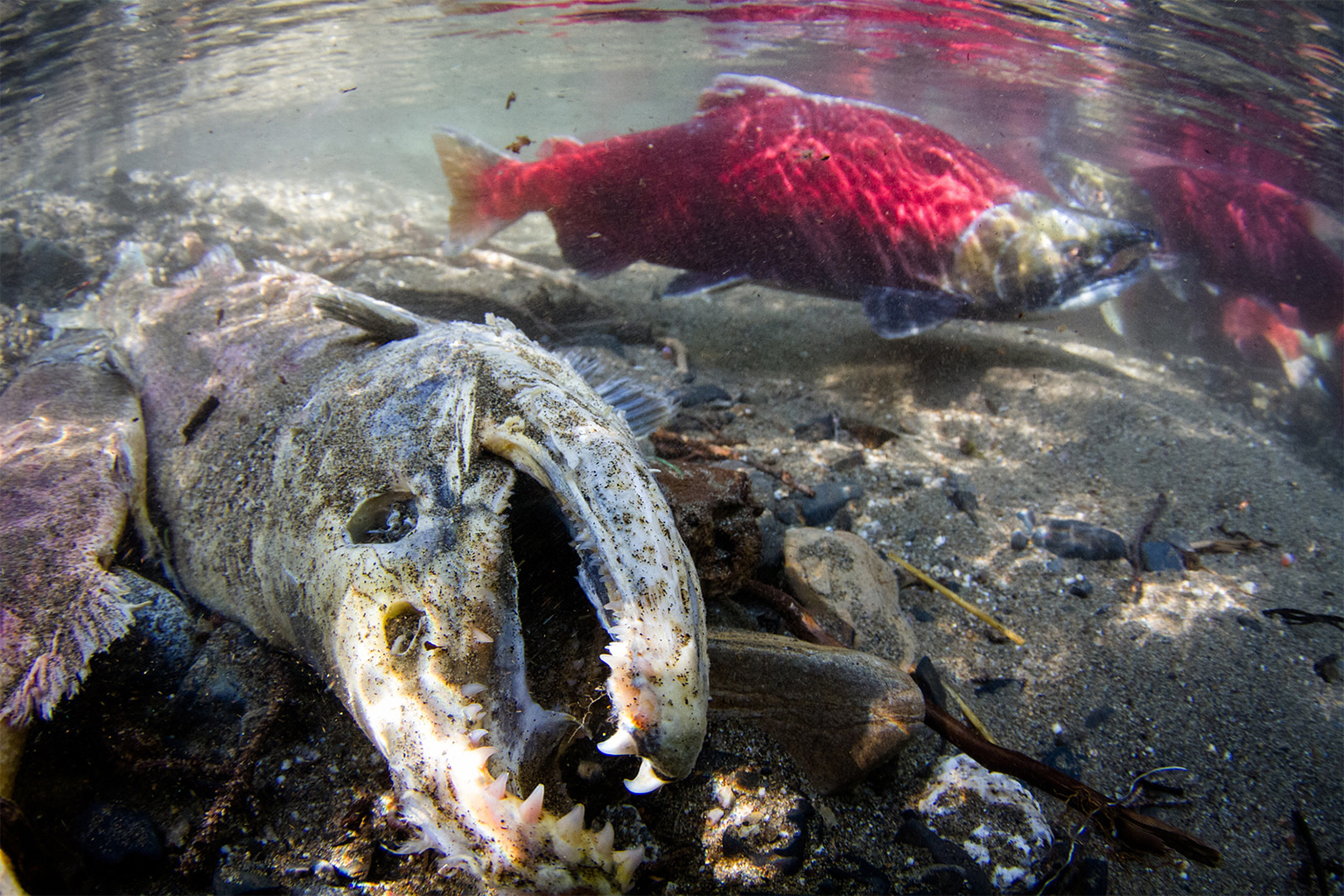
A matter of size
But in terms of the delivery of marine-derived nutrients, it’s not just the number of fish that matters; it’s size, too.
“If you talk to folks who handle a lot of fish — fishers or biologists that have handled fish in Alaska and other places over the last 20 to 30 years — they almost to a person will tell you the fish are smaller,” says Eric Palkovacs, professor of ecology and evolutionary biology at the University of California, Santa Cruz.
To confirm these anecdotal observations, and determine what it meant for ecosystems, Palkovacs and his team analyzed 60 years of fisheries data on four species of salmon across Alaska. Their findings, published in a 2020 study in Nature Communications, confirmed that all species had gotten smaller, by as much as 8% in the case of Chinook salmon. The most dramatic size declines happened since 2000.
But why were salmon getting smaller? Most likely because they are returning to spawn at a younger age — fewer years in the ocean means less time to grow — though the salmon also appear to be growing more slowly, scientists say.
“There isn’t one single smoking gun” as to why they are spawning earlier, says Palkovacs, but climate, fishing pressure and competition from hatchery salmon are all playing a part.
For Chinook the dramatic size decline equates to a 28% reduction in nutrient transport. That amounts to a sizeable loss for some ecosystems. Palkovacs likens the Yukon River chinook run — the 3,000-km journey that is the longest salmon run in the world — to “a highway of nutrients to make it all the way from the ocean into interior Alaska and even British Columbia.”
“Most fishery managers think about the numbers of fish. But the ecological processes and the value of the fish to the fishers also depends on the size of those fish,” Palkovacs explains.
Looking even further back, the change is even starker. According to a 2011 study published in Fisheries, only 5-7% of historic levels of marine-derived nitrogen and phosphorus are now available to ecosystems in the U.S. Pacific Northwest owing to dramatic salmon population declines following European contact. That loss may have “contributed to the downward spiral of salmonid abundance and diversity in general,” the authors wrote.
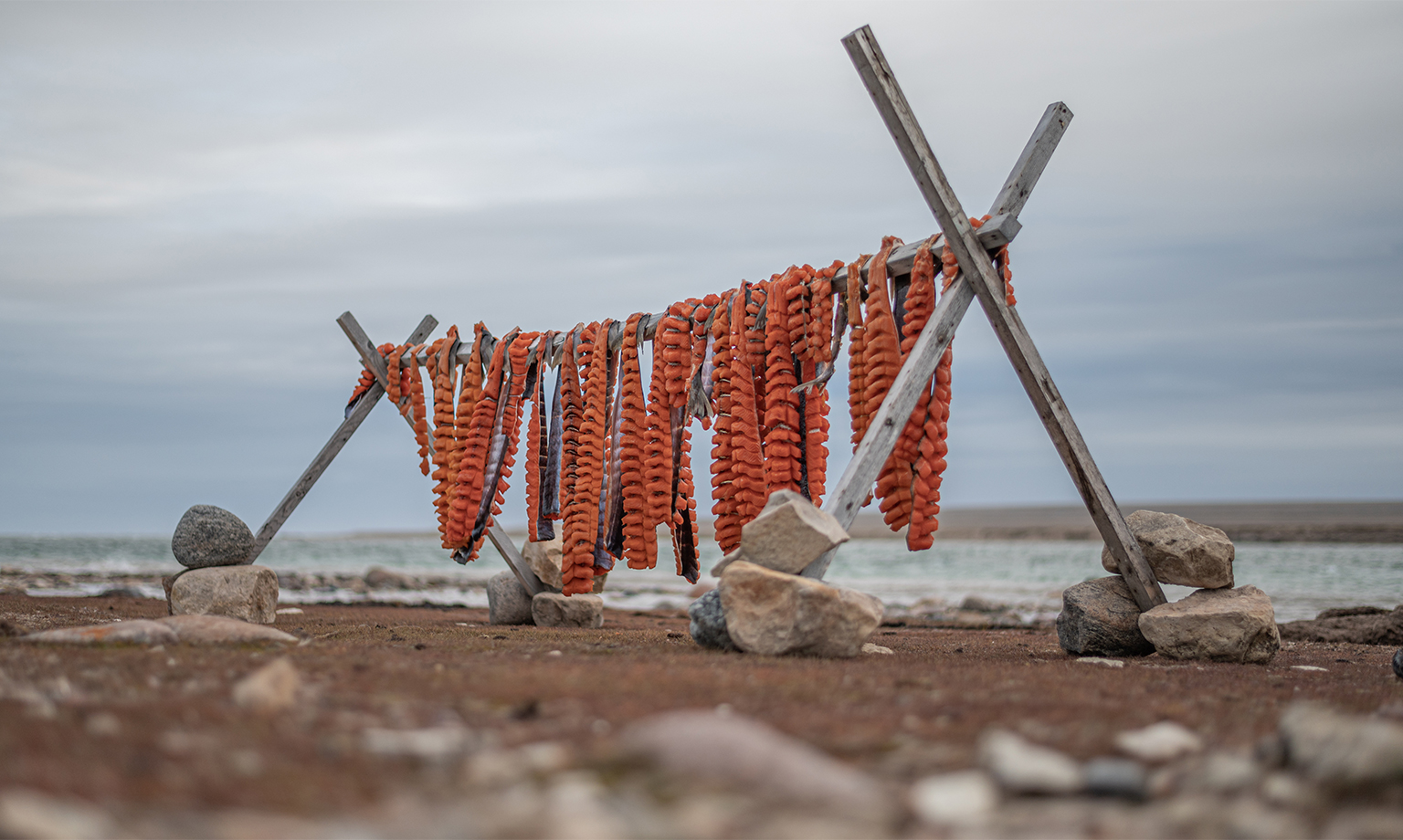
Other anadromous fish under pressure
While not studied as well, many other anadromous fish in the Arctic, Atlantic and around the world face similar pressures to Pacific salmon. Overall, populations of migratory fish that move between the ocean and freshwater have declined by 73% since 1970, according to a 2020 report by the World Migratory Fish Foundation; in fact, many populations had declined significantly even before this period, the report notes.
But it’s not so easy to figure out how those declines impact ecosystems in terms of nutrient cycling.
Sea-run Arctic char (Salvelinus alpinus) on Canada’s east coast, for example, live in a relatively pristine environment, but are highly impacted by climate change. “We’ve seen temperatures as high as 21°C [70°F] in Arctic rivers during the upper river migration period,” says Matthew Gilbert, assistant professor with the Institute of Arctic Biology at the University of Alaska, Fairbanks, who is studying how Arctic char respond physiologically to warming temperatures.
His research indicates that those high temperatures are lethal for char, even in the short term. “I would say that there are some systems where that may have already stopped migration in some years and may ultimately result in the inability of fish in those populations to complete their migration at all,” he says. Instead, some may end up forgoing migration entirely, and remain in freshwater lakes year-round.
Sea-run Arctic char take in marine-derived nutrients when they feed in the ocean during the summer. Those nutrients enter the terrestrial ecosystems when the char deposit their eggs in September and October, and excrete feces and urine, and when they’re eaten throughout the year. Because Arctic lakes are oligotrophic, meaning they don’t have a lot of nutrients, theoretically even small additions may have an outsized effect, though so far studies have found limited evidence of this.
Another example: Alewife (Alosa pseudoharengus), a type of anadromous river herring found in the northwest Atlantic that today are found in highly altered landscapes. In the eastern U.S., populations have been hit hard by the construction of hydroelectric dams.
As alewife populations declined dramatically, so did the amount of marine-derived phosphorus and nitrogen they delivered to freshwater ecosystems, according to a 2017 BioScience study. But at the same time, there’s been an increase in phosphorus and nitrogen in those ecosystems due to pollution and fertilizer runoff, so the overall impact of that loss isn’t clear.
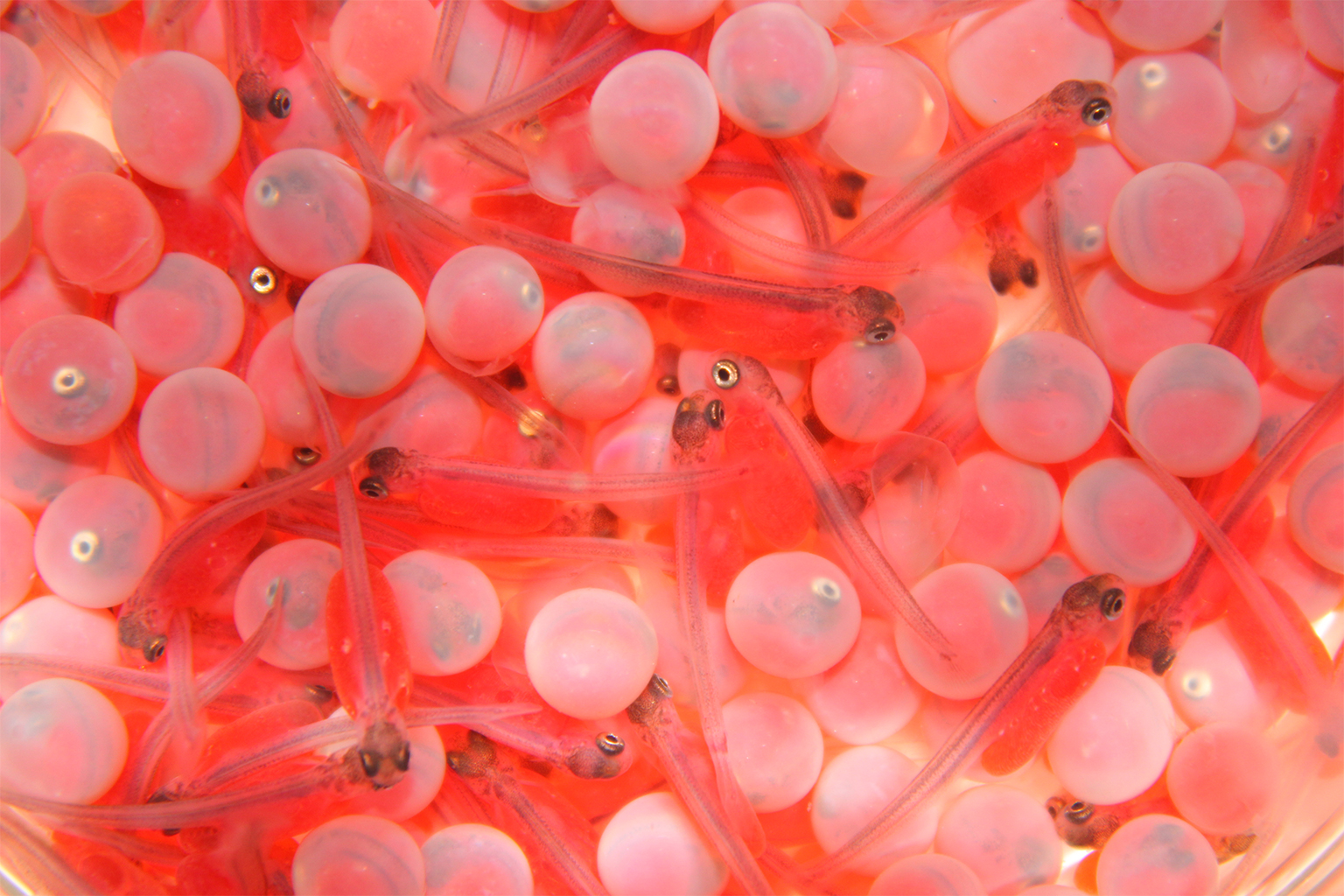
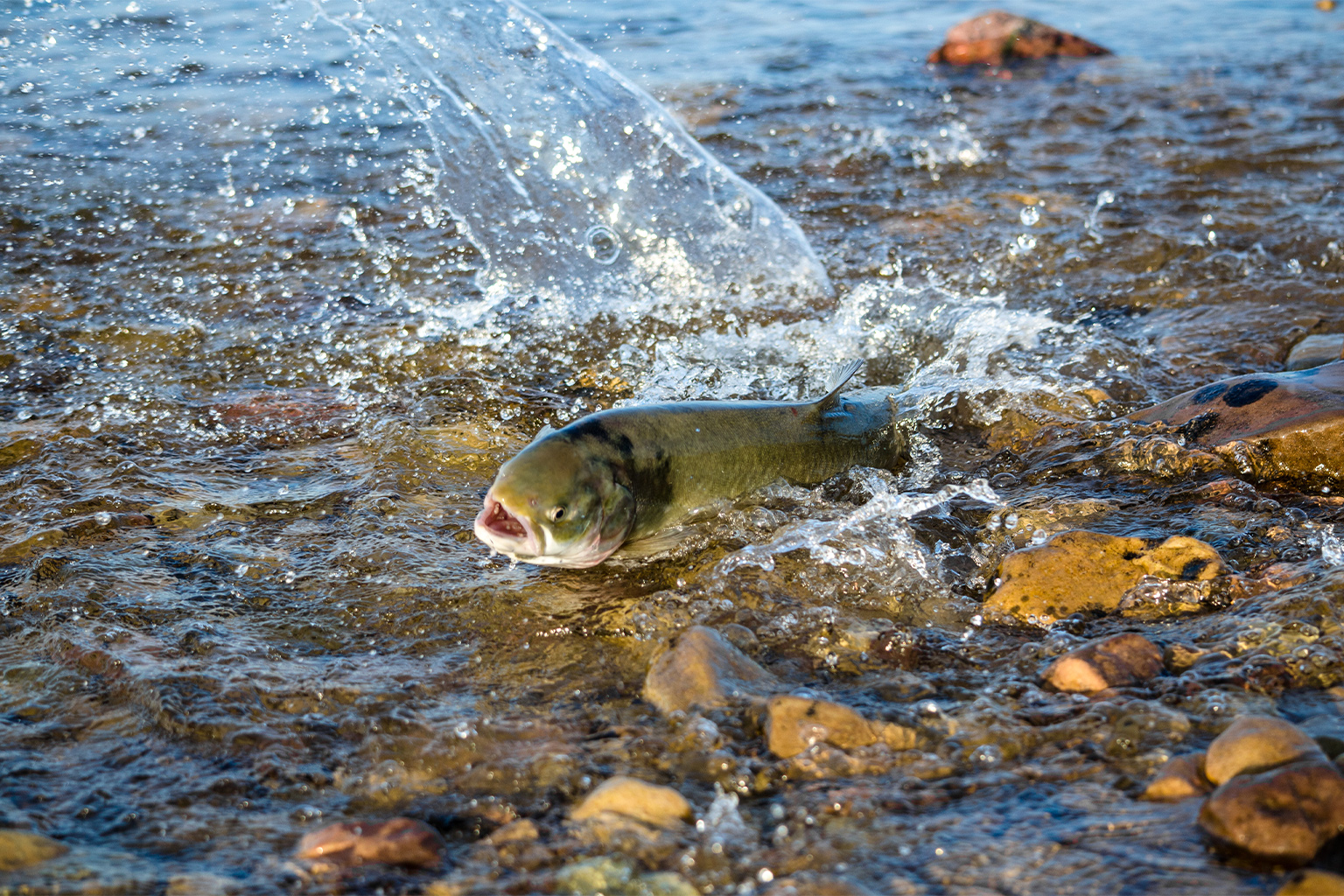
A way forward: Reviving biological connectivity
What is clear is that wildlife and far-flung ecosystems don’t operate in isolation. They are connected through complex biological processes that underpin planetary health.
In a 2022 paper published in Science of The Total Environment, Doughty and colleagues proposed revitalizing the world’s “natural phosphorus pump” by restoring wildlife populations and reestablishing connections between marine, terrestrial and freshwater ecosystems. This could augment other strategies to recycle phosphorus.
“We’re just getting a ton of phosphorus out of the ground, and we’re flushing it to the bottom of the ocean,” Doughty says. “But if we restore some of these cycles — salmon are a great example of that — you can move some of the phosphorus back on the land. That’s a real value.”
For Pacific salmon and other anadromous fish to regain their full nutrient transport role, that would mean restoring a diversity of freshwater habitats, removing barriers to migration, ensuring healthy oceans, alleviating fishing pressure, and mitigating other pressure, while making sure salmon have the space and genetic capacity to adapt to climate change.
“The best thing we can do” for Pacific salmon, says Moore from Simon Fraser University, “is get out of their way.”
Banner image: A black bear fishes for salmon. When bears carry salmon into the nearby forests to feed, the carcasses are scavenged by other creatures, and eventually the nutrients are incorporated into riparian ecosystems. Image by Jonny Armstrong courtesy of the Salmon Science Network.
Citations:
Abraham, A. J., Roman, J., & Doughty, C. E. (2022). The sixth R: Revitalizing the natural phosphorus pump. Science of The Total Environment, 832, 155023. doi:10.1016/j.scitotenv.2022.155023
Cheung, W. W., & Frölicher, T. L. (2020). Marine heatwaves exacerbate climate change impacts for fisheries in the northeast Pacific. Scientific Reports, 10(1), 6678. doi:10.1038/s41598-020-63650-z
Dennert, A. M., Elle, E., & Reynolds, J. D. (2023). Experimental addition of marine-derived nutrients affects wildflower traits in a coastal meta-ecosystem. Royal Society Open Science, 10(1), 221008. doi:10.1098/rsos.221008
Doughty, C. E., Roman, J., Faurby, S., Wolf, A., Haque, A., Bakker, E. S., … Svenning, J. C. (2016). Global nutrient transport in a world of giants. Proceedings of the National Academy of Sciences, 113(4), 868-873. doi:10.1073/pnas.1502549112
Gresh, T., Lichatowich, J., & Schoonmaker, P. (2000). An estimation of historic and current levels of salmon production in the Northeast Pacific ecosystem: Evidence of a nutrient deficit in the freshwater systems of the Pacific Northwest. Fisheries, 25(1), 15-21. doi:10.1577/1548-8446(2000)025<0015:AEOHAC>2.0.CO;2
Kieran, C. N., Obrist, D. S., Muñoz, N. J., Hanly, P. J., & Reynolds, J. D. (2021). Links between fluctuations in sockeye salmon abundance and riparian forest productivity identified by remote sensing. Ecosphere, 12(8), e03699. doi:10.1002/ecs2.3699
Mattocks, S., Hall, C. J., & Jordaan, A. (2017). Damming, lost connectivity, and the historical role of anadromous fish in freshwater ecosystem dynamics. BioScience, 67(8), 713-728. doi:10.1093/biosci/bix069
Oke, K. B., Cunningham, C. J., Westley, P. A. H., Baskett, M. L., Carlson, S. M., Clark, J., … Palkovacs, E. P. (2020). Recent declines in salmon body size impact ecosystems and fisheries. Nature Communications, 11(1), 4155. doi:10.1038/s41467-020-17726-z
Richardson, K., Steffen, W., Lucht, W., Bendtsen, J., Cornell, S. E., Donges, J. F., … Rockström, J. (2023). Earth beyond six of nine planetary boundaries. Science Advances, 9(37), eadh2458. doi:10.1126/sciadv.adh2458
Siemens, L. D., Dennert, A. M., Obrist, D. S., & Reynolds, J. D. (2020). Spawning salmon density influences fruit production of salmonberry (Rubus spectabilis). Ecosphere, 11(11), e03282. doi:10.1002/ecs2.3282
Swain, N. R., & Reynolds, J. D. (2015). Effects of salmon-derived nutrients and habitat characteristics on population densities of stream-resident sculpins. PLOS One, 10(6), e0116090. doi:10.1371/journal.pone.0116090
Tian, Z., Zhao, H., Peter, K. T., Gonzalez, M., Wetzel, J., Wu, C., … Kolodziej, E. P. (2021). A ubiquitous tire rubber–derived chemical induces acute mortality in coho salmon. Science, 371(6525), 185-189. doi:10.1126/science.abd6951
Wilcox, K. A., Wagner, M. A., & Reynolds, J. D. (2021). Salmon subsidies predict territory size and habitat selection of an avian insectivore. PLOS One, 16(7), e0254314. doi:10.1371/journal.pone.0254314
Wilson, S. M., Moore, J. W., Ward, E. J., Kinsel, C. W., Anderson, J. H., Buehrens, T. W., … Wyatt, G. J. (2023). Phenological shifts and mismatch with marine productivity vary among Pacific salmon species and populations. Nature Ecology & Evolution, 1-10. doi:10.1038/s41559-023-02057-1
FEEDBACK: Use this form to send a message to the author of this post. If you want to post a public comment, you can do that at the bottom of the page.