- We know that soil feeds plants, but do we know how it got there in the first place? Soil forms via the interaction of five factors: parent material, climate, living beings, a land’s topography, and a “cooking” time that occurs on a geologic scale. Variations in these 5 factors make the world’s soils unique and extremely diverse.
- Soil acts as a carbon sink in the global carbon cycle because it locks away decomposed organic matter. But deforestation, various agricultural practices, and a changing climate are releasing it back into the atmosphere and oceans as carbon dioxide, resulting in an imbalance in global carbon budgets
- Tropical soils and permafrost hold the most soil carbon out of other biomes, making them conservation and research priorities in soil-centered climate solutions.
- Reforestation of previously forested lands is a viable solution to return carbon belowground, but it is not a fix-all. Changing industrial agricultural practices and giving high-carbon storage areas conservation status are key steps toward harnessing the soil’s carbon storage power.
We know soils feed plants, and plants feed us, but the world’s soils do more than help put food on the table. Soils recycle nutrients, regulate our water supply and, importantly for this moment in history: they store more carbon than plants, animals and the atmosphere combined. So, focusing humanity’s restoration efforts belowground could turn out to be an essential piece of the climate change solution puzzle.
“Most signs point to ongoing climate change leading to losses of ecosystem carbon stocks,” said Daniela Cusack, an assistant professor at Colorado State University and expert in tropical soil biochemistry. It’s really important that “we restore that natural pathway of carbon into the soil.”
But, do we really know what soils are, how they form, how they get degraded and then repaired, and what they can do to support ecosystems and humanity? A dive below ground can help explain how a soil-focused solution to our climate change problems might work.
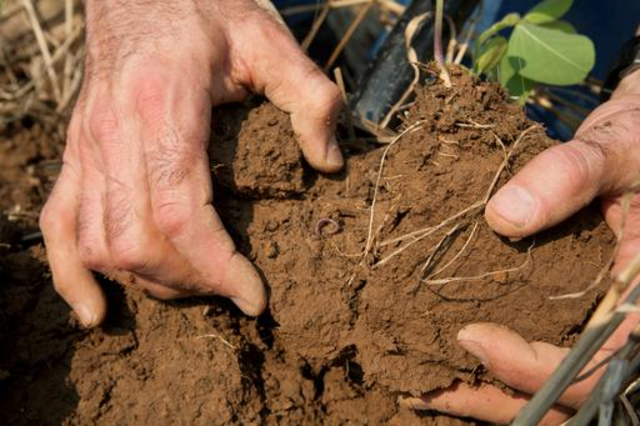
The basics: Getting to know the dirt on dirt
First off, soil is made via the interaction of five soil-forming ingredients: parent material, climate, living beings, the unique topography of a place, and a “cooking” time that occurs on a geologic scale.
Soil begins to form when rocks are broken apart by a process called weathering. Rocks can be broken down physically via alternating periods of hot and cold, or by abrasion from wind, water or ice. Rocks can also be weathered chemically by acids made by microbes and plants, or when water and oxygen interact with minerals bound in the rock.
The products of weathering are what scientists call parent material. Minerals from rock, such as magnesium and iron, or organic materials, such as decayed vegetation, are free to interact with the environment, becoming the precursor of soil. A soil’s parent material can come from the rock directly beneath it, or it can be transported by water or wind from one location and deposited in another. Parent material can have multiple origins and figuring out what they are and their mode of transport is a crucial part of understanding a soil’s unique history.
Climate is also a vital ingredient in soil formation. By controlling temperature and precipitation over large areas, it determines how weathering occurs. Hot, moist environments like tropical rainforests, for example, have some of the most weathered soils on Earth, and that’s largely due to climate.
“Tropical soils have had a long time for weathering to occur because, unlike temperate systems, they did not have a glaciation period that scraped the soil away when the glaciers retreated,” Cusack tells Mongabay. Many tropical soils have eluded the glacial reset button and never been frozen, making them some of the most weathered and deepest soils on Earth.
In addition, climate influences the kinds of plants and animals that can occupy an area. These living beings, another ingredient, affect soil formation within their lifetimes, but also after. Living plants take up and release nutrients back into the soil, while animals like earthworms and ants make burrows, creating pathways for air, water and nutrients to move through. When living beings die, they’re transformed by microbes into organic matter, namely carbon. Some of that carbon accumulates in the soil and interacts with minerals made from parent material, further building the soil.
The topography of a region, or the lay of the land, can speed up or slow down weathering. Topography influences where plants grow and how water moves through the landscape, helping determine where parent material builds up and where rock remains protected from erosion.
Considering parent material, climate, living beings and topography in the context of time, the final ingredient, we see that soil formation is an act of destruction and synthesis. All five of the soil-forming elements work simultaneously and independently over long time scales, creating a mosaic of soil types with unique histories and properties.
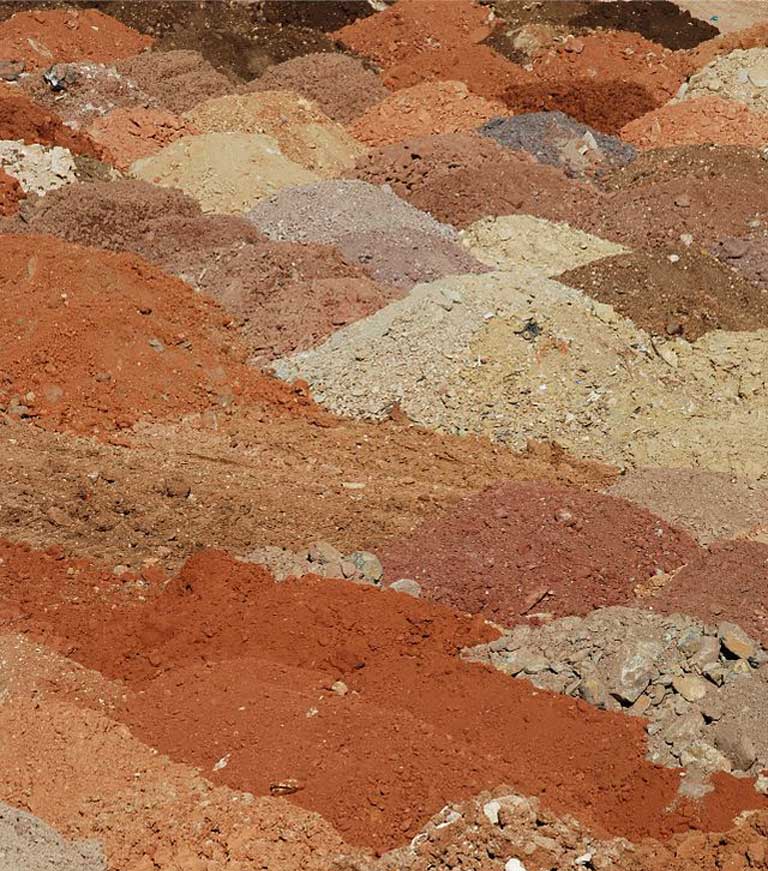
To understand a soil in a particular place, scientists first look at the distinct layers of soil belowground, called horizons. Each horizon tells a story of events in Earth’s past, and reveals how the five soil-forming factors interacted in that area.
Every soil has a sequence of horizons, like layers in a cake, and the vertical arrangement of the horizons is called the soil profile. Scientists dig into these soil profiles to expose the horizons and study their properties — what gives the soil its texture, holds its structure, and supports its life: mineral particles, organic matter, water, air and microbes. The types and proportions of these properties determine what a soil can do in its environment (what it can grow, for example, or how much carbon it can hold).

Sand, silt and clay particles derived from parent materials act as the soil’s skeleton, providing a solid medium in which water, air and microbes can exist and also move. Clay minerals are the smallest particles but play an important role in soil function. They’re shaped like tiny flakes or sheets with many facets, giving them large surface areas with a tremendous capacity to hold onto nutrients, water and carbon, like a magnet. Their carbon-binding capability is one of the reasons why undisturbed soils can hold so much carbon for such a long time.
Soil and its role in the carbon cycle
Understanding how soil works and what it is made of is crucial to understanding the global carbon cycle, and climate change. The fate of organic matter in the soil, for example, connects soils to both the atmosphere and the ocean (the other major global carbon sinks). But how does carbon dioxide relate to the carbon stored in the soil?
To answer this question, we look to the plant kingdom. When plants make their own food via photosynthesis, they act as carbon dioxide straws, absorbing CO2 from the atmosphere and transforming it into wood, roots and leaves.
“Plants are responsible for getting the carbon into the soil in the first place,” Cusack explained. Trees, in particular, remove large amounts of carbon dioxide from the atmosphere and serve as natural buffers to greenhouse gas-induced climate change.
When plants, or plant parts, and other organisms die they become organic matter. Microbes in the soil release enzymes that break those organisms down into reusable nutrients and smaller carbon compounds. Some of those carbon compounds feed other soil organisms, some accumulate in the soil and become attached to the soil skeleton, and some are exhaled as carbon dioxide into the atmosphere and then absorbed by the ocean, connecting soils to the global carbon cycle.
When carbon is held in the soil, it becomes sequestered, or locked away, and it can remain stable there for millions of years if undisturbed. This is why soil is called a carbon sink.

Soil carbon sink contrasts: From the Tropics to the Arctic
Because of climate, different biomes store varying amounts of soil carbon. “Tropical soils and sub-Arctic permafrost soils store the most carbon, but in really different ways,” Cusack told Mongabay. “The deep soils of the tropics with a lot of surface area on their clays have locked carbon in, and what was originally root carbon [in the plant] has been transferred down through the soil profile [into the soil].”
Cusack and her colleagues at Lawrence Livermore National Lab in California have been using radiocarbon dating to estimate the average age of the carbon in tropical soils. “Carbon that has been absorbed onto clay surfaces is the oldest, like a million years old in some sites,” she said.
Way up North, by contrast, permafrost soils have been frozen for thousands of years, so plant biomass deposited there has not decomposed and has remained frozen in time, representing a really large pool of terrestrial carbon. “Organic matter is frozen so deep down, that there is no turnover of carbon. It’s just frozen in the soil,” Cusack said.
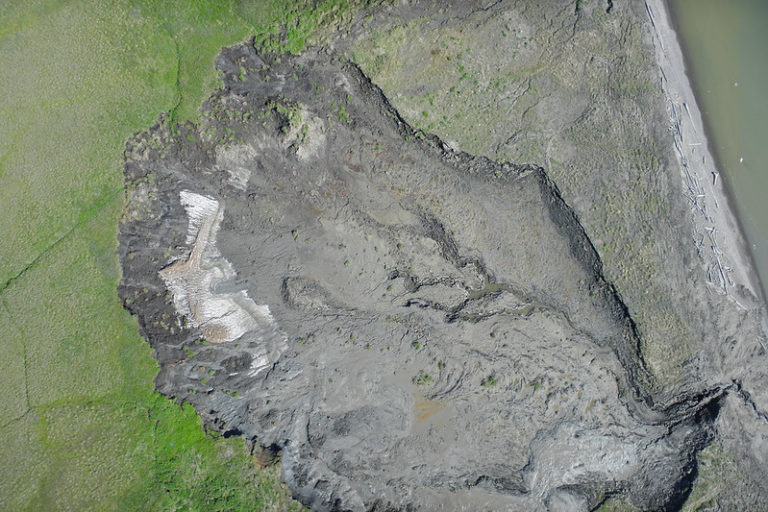
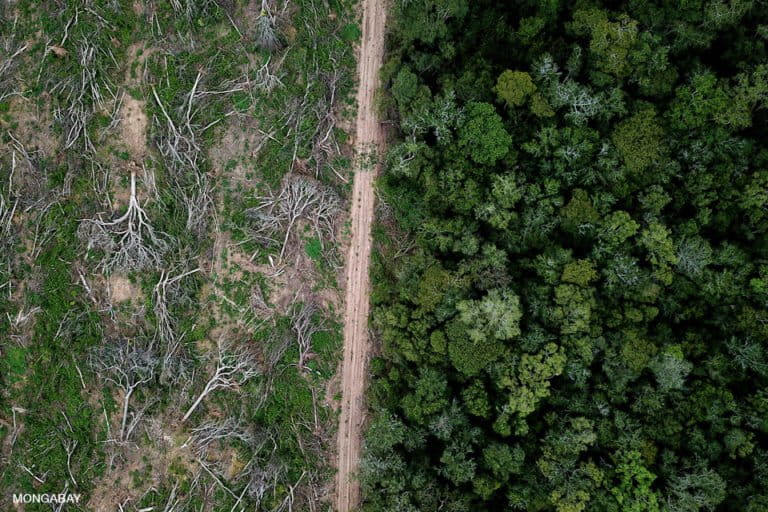
Climate change altering soil carbon storage
Climate change is being accelerated as humanity contributes high amounts of carbon dioxide and other greenhouse gases to the atmosphere.
Widespread deforestation, particularly in tropical forests, is removing plants — the connection between the atmosphere and the soil. Largescale removal of forests means that carbon dioxide accumulates in the atmosphere instead, causing an imbalance in the global carbon budget and altering Earth’s climate patterns.
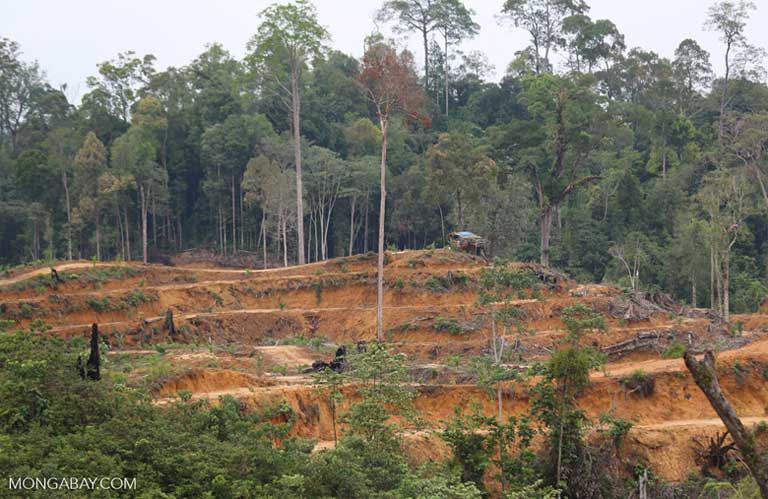
Further, invasive agricultural practices and land use changes — such as tillage that uses aggressive plowing techniques, or disrupting wetland drainage — are digging up and breaking up the soil profile and exposing sequestered soil carbon to the air. This disturbance causes the carbon compounds to react with oxygen, making it rise from its stable sleep belowground to become carbon dioxide expelled skyward.
Carbon dioxide in the atmosphere has been linked to an increase in Earth’s temperatures. Permafrost soils, for example, are particularly feeling the heat, and they are thawing in unexpected ways. “Frozen soil doesn’t just lock up carbon — it physically holds the landscape together,” writes Merritt R. Turetsky, associate professor at the University of Colorado-Boulder, in a comment to Nature.

But now, “Permafrost is collapsing suddenly as pockets of ice within it melt. Several meters of soil can become destabilized within days or weeks,” Turetsky explained. Permafrost melt, triggered by human-caused global warming, is also causing thawed sub-Arctic and Arctic soils to release methane to the atmosphere — posing a huge climate threat because methane is many times more powerful as a greenhouse gas than carbon dioxide. “Understanding abrupt thawing must be a research priority,” writes Turetsky.
Meanwhile, in the tropics, more carbon dioxide in the atmosphere means more warming and more intense dry seasons, which rainforests aren’t used to. Cusack is the lead principal investigator of a new, long-term study about how drying affects plants and soil carbon stocks. Her team has put up structures that reduce rainfall to a small portion of the tropical rainforest floor by 50%. They are finding that sites that naturally get a lot of rainfall are more negatively impacted when rainfall is reduced, while naturally dry sites do better because they’re already used to dry conditions, so they may be able to adapt to longer droughts in the future.
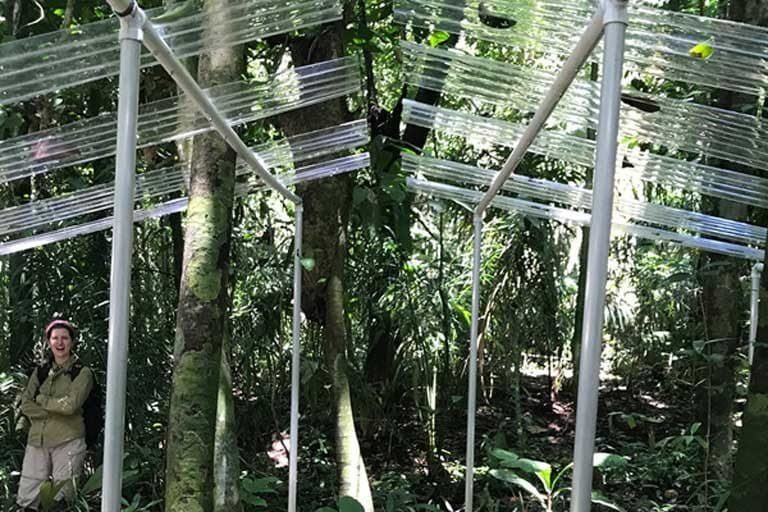
Drying also affects the age of the soil carbon being released. “In the [drier] experiment plots, we are losing more old soil carbon, whereas, in our control plots, the carbon is younger and more reflective of recent root and litter inputs,” said Cusack. This means drying is causing the sequestered or “locked away” carbon to be released.
“If this was sort of generalized across the tropics,” Cusack said, “we would be really concerned that we are losing this older soil carbon.”
Soil solutions 101
Top scientists agree that carbon soil storage could be key to solving our climate conundrum. The vital question is: How do we maintain existing soil carbon, while also getting atmospheric carbon sequestered back into soils?
Reforestation of previously forested land is a viable climate change-curbing solution because it adds carbon back to the soil as young seedlings grow into mature trees. “The roots are putting the carbon in the soil. But that first step of getting really extensive root systems in the soil is really important” Cusack said.
But reforestation is not a fix-all for every biome that is losing soil carbon. Also, the type of restoration matters: reforestation via tree plantations, for example, won’t help much, because fast-growing trees that are soon cut to become toilet paper or forest biomass converted to wood pellets that are burned to make energy, don’t sequester carbon. Importantly, forests are not individual trees but complex, connected systems that are built over time. Carefully recreating natural long-growing forests could provide a significant climate solution.

Another solution would give high-carbon storage areas legal conservation status. Initiatives like the United Nations’ REDD+ program work in that direction by providing economic and technical support to developing countries and communities to help them conserve already existing forests. Conservation of soil carbon stocks is a central goal of REDD+, a largely voluntary, incentive-based program, and it is working with some success in the tropics. Creating new state-protected carbon storage reserves could also be a winning strategy.
But in the case of preserving melting permafrost, neither conserving land nor planting native vegetation will stop escalating greenhouse gas releases. For the moment, drastically and quickly reducing humanity’s global carbon emissions seems the sole best way of keeping sub-Arctic carbon and methane underground.

Changing agricultural practices worldwide could also protect soils and greatly enhance their carbon storage capacity. According to many experts, industrial agribusiness needs a complete overhaul, moving away from synthetic fertilizers (which wreck the nitrogen and phosphorous balance), reducing the use of pesticides (which ravage soil biodiversity), and implementing no-till or low-till sustainable practices that build resilient soils for the long haul.
Still, we have much to learn, and certainly, a well-funded worldwide soils study program is urgently needed. Understanding soil origins, properties, capabilities and time scales — and how we’re altering them — could help heal our strained relationship with the earth, and be a pivotal step toward harnessing soil’s carbon storage power to limit climate change.
Restoring Earth’s carbon balance requires a menu of solutions at many scales, but soil is at the center of them all.
Citations:
Turetsky, M.R., Abbott, B.W., Jones, M.C., Anthony, K.W., Olefeldt, D., Schuur, E.A., Koven, C., McGuire, A.D., Grosse, G., Kuhry, P., Hugelius, G., Lawrence, D.M., Gibson, C., Sannel, A.B.K. (2019). Permafrost collapse is accelerating carbon release. Nature 569, 32-34. doi: https://doi.org/10.1038/d41586-019-01313-4
Stips, A., Macias, D., Coughlan, C. Garcia-Gorriz, E., San Liang, X. (2016). On the causal structure between CO2 and global temperature. Scientific Reports 6, 21691. https://doi.org/10.1038/srep21691
2021, May 25. UN-REDD+. https://www.un-redd.org
Banner image by Nestor T. via Flickr (CC BY NC 2.0)
FEEDBACK: Use this form to send a message to the author of this post. If you want to post a public comment, you can do that at the bottom of the page.