- Stripping seawater of carbon dioxide via electrochemical processes — thereby prompting oceans to draw down more greenhouse gas from the atmosphere — is a geoengineering approach under consideration for largescale CO2 removal. Several startups and existing companies are planning projects at various scales.
- Once removed from seawater, captured carbon dioxide can be stored geologically or used commercially by industry. Another electrochemical method returns alkaline seawater to the oceans, causing increased carbon dioxide absorption over time.
- In theory, these techniques could aid in carbon emission storage. But experts warn that as some companies rush to commercialize the tech and sell carbon credits, significant knowledge gaps remain, with potential ecological harm needing to be determined.
- Achieving the scale required to make a dent in climate change would require deploying huge numbers of electrochemical plants globally — a costly and environmentally risky scenario deemed unfeasible by some. One problem: the harm posed by scale-up isn’t easy to assess with modeling and small-scale projects.
Ocean-based carbon dioxide removal technology is ramping up, with startups and existing companies racing to develop electrochemical techniques that either remove carbon from seawater or prompt oceans to suck up more.
Electrochemical marine carbon dioxide removal (mCDR) aims to store carbon in the ocean or strip it from seawater using electricity.
Broadly speaking, mCDR is divided into two methods with some overlap: ocean alkalinity enhancement (OAE) and direct ocean capture (DOC), also known as direct ocean removal.
OAE electrochemically splits seawater into a base and acid stream. The base stream is added back to the ocean, notching up pH to make the ocean’s surface more alkaline, prompting more CO2 to be absorbed from the atmosphere over time in the form of bicarbonate. The acidic stream can be used by industry or sequestered in the deep sea.
“If you think of the ocean like a sponge, adding alkalinity makes that sponge bigger, so it’s able to take up more carbon dioxide,” says Helen Findlay at the Plymouth Marine Laboratory.
DOC also uses electrochemical processes to strip carbon dioxide from seawater. One method converts dissolved inorganic carbon within seawater into a gas that is then captured. Low CO2 water is returned to the ocean, where it naturally rebalances as carbon dioxide is drawn down from the atmosphere. The captured carbon can be stored geologically or used in industrial processes, much like direct air capture on land.
“Back to the sponge analogy, direct ocean capture is like squeezing the sponge and then allowing it to soak back up again,” Findlay says.
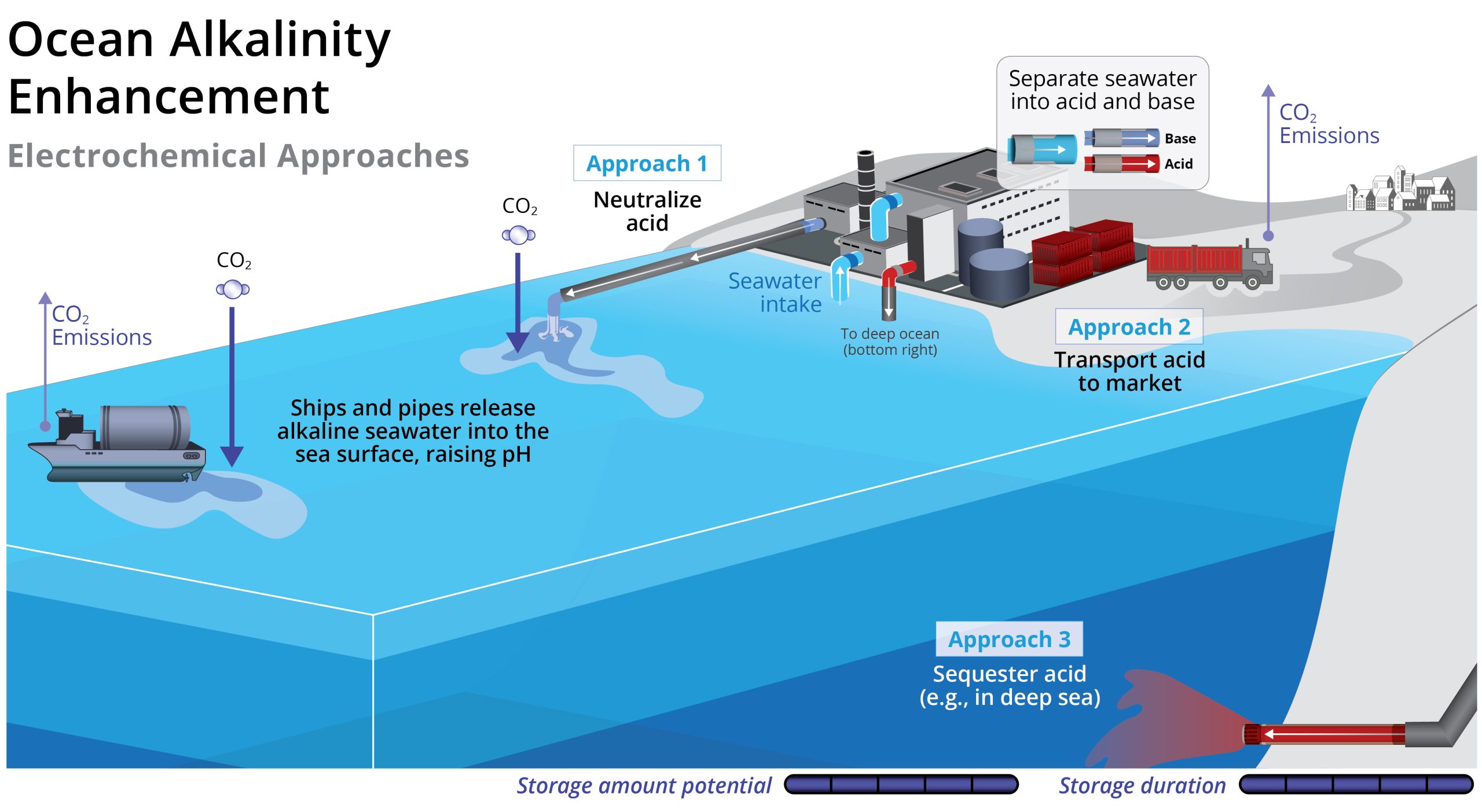
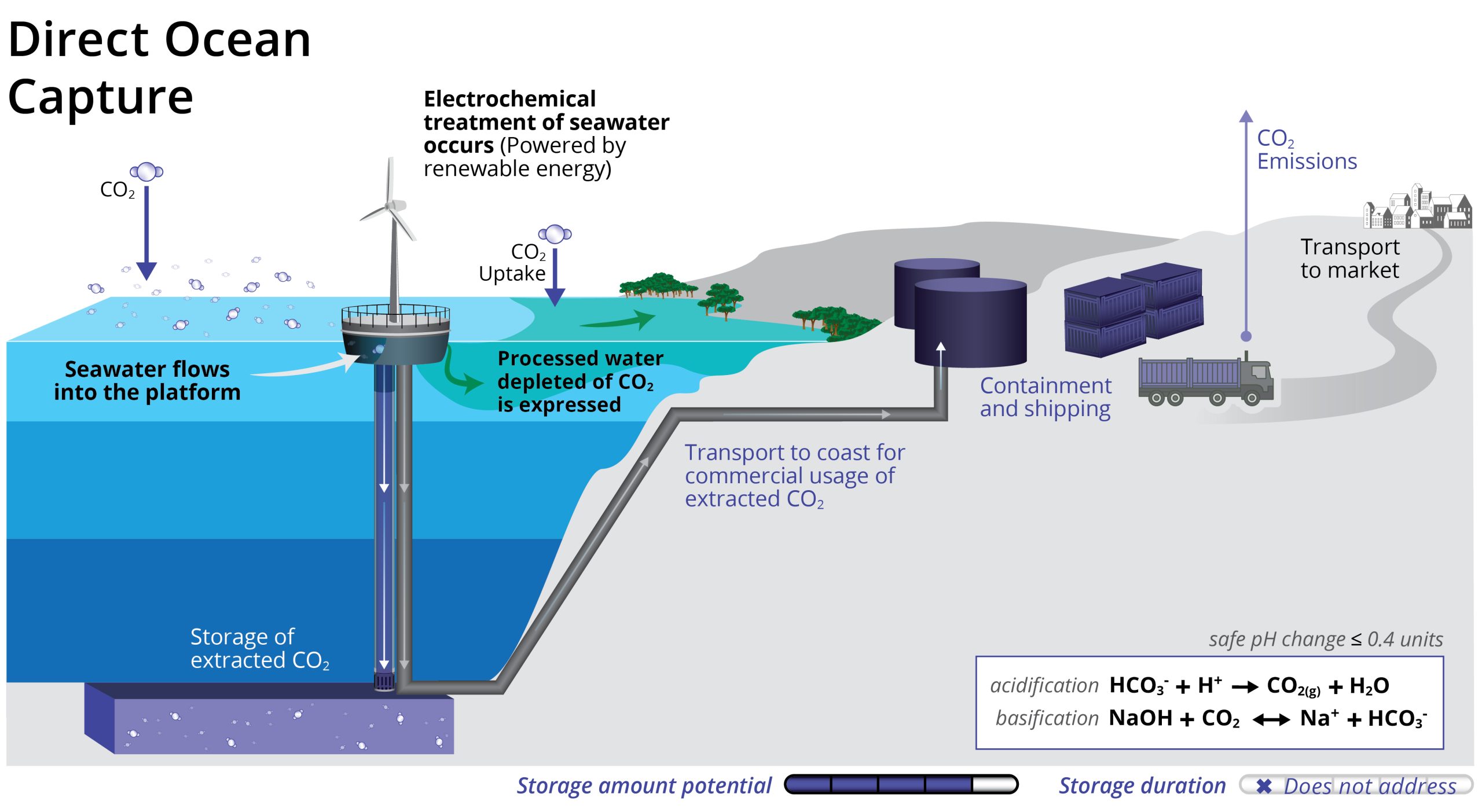
Several pilot projects and trials that utilize variations of these approaches are either planned or underway globally, with the companies involved helping pay for their work by selling carbon credits.
That’s bringing significant investment from corporations such as Microsoft, which recently signed a deal with U.S.-based OAE company Ebb Carbon, securing a future option to purchase more than 300,000 tons worth of carbon credits. U.S.-based Captura, meanwhile, plans to develop a 1,000-ton plant in Hawaii next year to “fast track” commercialization. Companies in Europe are planning their own small-scale tests.
U.S.-based Equatic plans to build a pre-commercial plant in Singapore as early as next year and add a commercial-scale plant in North America in 2026. The latter will be the world’s largest mCDR facility to date and is projected to remove more than 100,000 tons of CO2 per year. That effort builds on the back of two 100-ton pilot plants in Singapore and Los Angeles that the firm has operated for more than a year.
“The ocean is the largest carbon sink on the planet and spans the entire globe, making it an accessible means for removal,” says Equatic chief operating officer Edward Sanders. “Our goal is to achieve gigaton-scale carbon removal, and we’re making critical progress across the world.”
But this frenzied pace of development and the drive to commercialization are causing concern among some analysts who fear science is lagging behind industry ambitions.
Though the theory behind both processes is well understood, achieving mCDR in practice remains largely unproven. Myriad questions remain about potential environmental impacts and efficacy for scaled-up mCDR, say experts such as Findlay.
“I think moving to actually implement these in a commercial way is not responsible yet, especially if we start to sell carbon credits on the back of them,” Findlay explains. “I don’t think we’re in a position to really say yes or no that they are even working correctly.”
At present, no ocean-based carbon dioxide removal (CDR) tech is ready for deployment, agrees Scott Doney, an ocean chemist at the University of Virginia who co-authored a 2022 research strategy for ocean carbon dioxide removal, including electrochemical approaches. “But given that it’s going to take a long time to scale these [methods] up, and [given that] we don’t have a huge amount of time … to address the climate change crisis, we need to be doing research now,” he says.
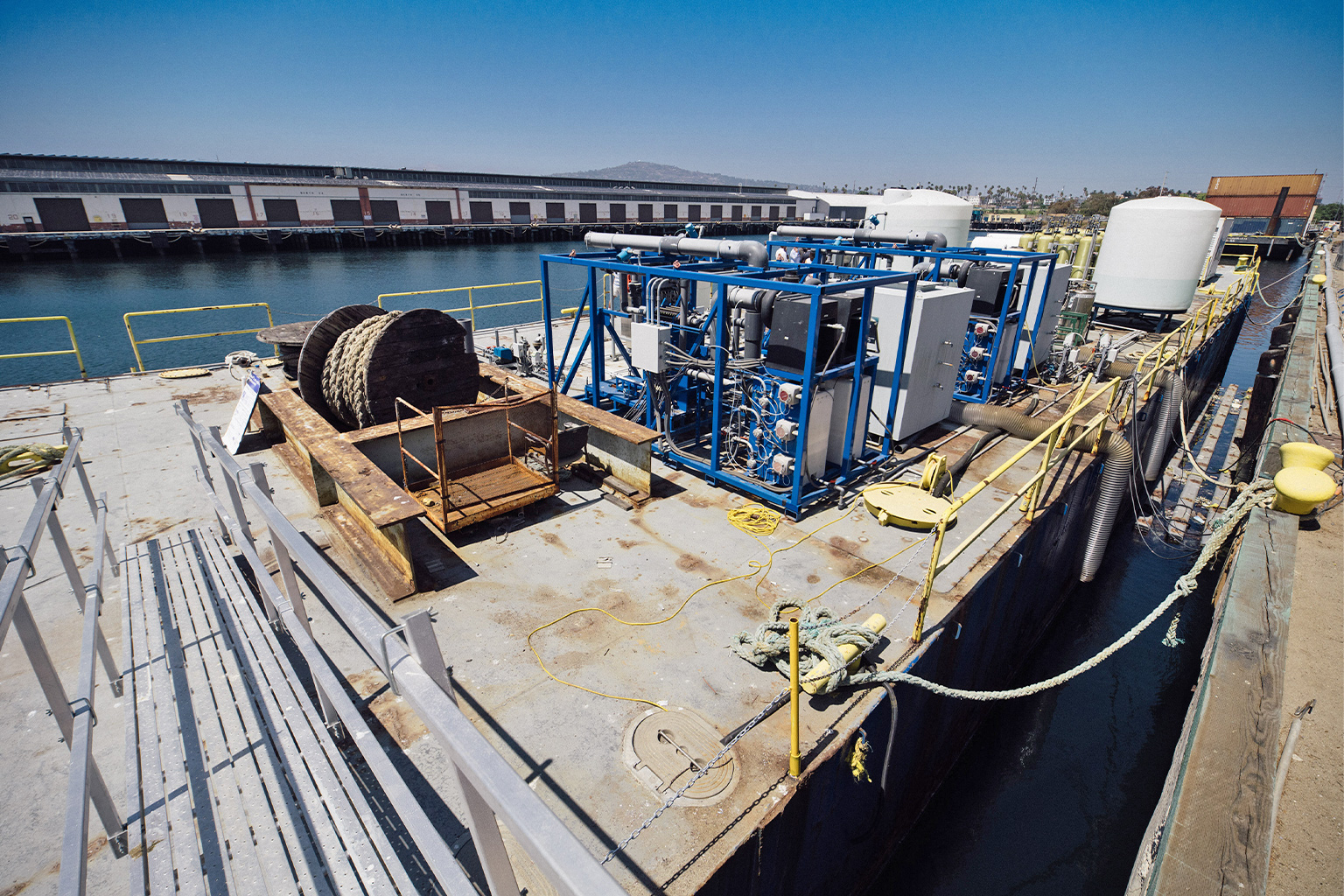
Playing with ocean chemistry
Scientists largely agree that, in addition to drastically cutting fossil fuel extraction and carbon emissions, humanity must remove billions of tons of CO2 already accumulated in the atmosphere to prevent catastrophic climate change. But how best to do it?
Like other types of CDR, marine electrochemical methods are hyped as having huge carbon removal potential at the gigaton scale. But unlocking that potential is no simple task, with each method faced with trade-offs, huge logistical challenges and the need for proof of concept upon which to build a workable business model.
Once smaller projects are well established, scale-up poses the next level of challenge for would-be electrochemical mCDR pioneers.
And scaling up is where things get really daunting: Researchers at UCLA have estimated that sequestering 10 billion tons of atmospheric CO2 per year via oceanic direct capture will require around 1,800 facilities worldwide, costing trillions of dollars.
In the near term, some companies envisage attaching their electrochemical tech to desalinization plants that already take in vast sums of seawater. Or making use of decommissioned oil rigs, in the case of U.S.-based Captura. Others, such as Equatic, plan to sell co-products made during the mCDR process, such as hydrogen. These strategies could cut the projected high costs of removing carbon and help pay for scaling tech.
But these high hopes all hinge on accurate monitoring, reporting and verification (MRV); all crucial if firms are going to pay for their projects by selling credits on carbon markets. Currently, both OAE and DOC are plagued by myriad scientific uncertainties in determining how much manipulated carbon is absorbed by oceans.
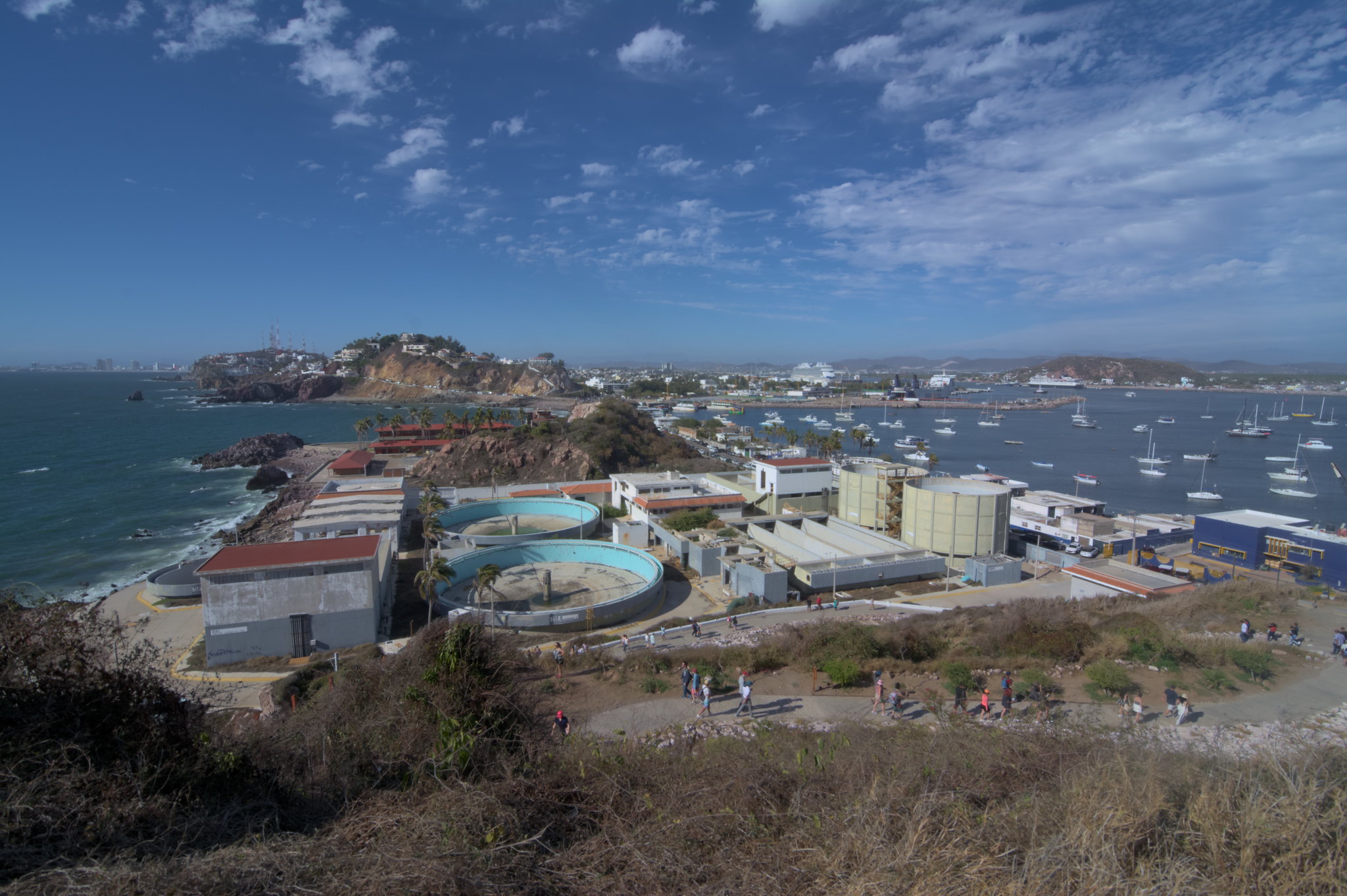
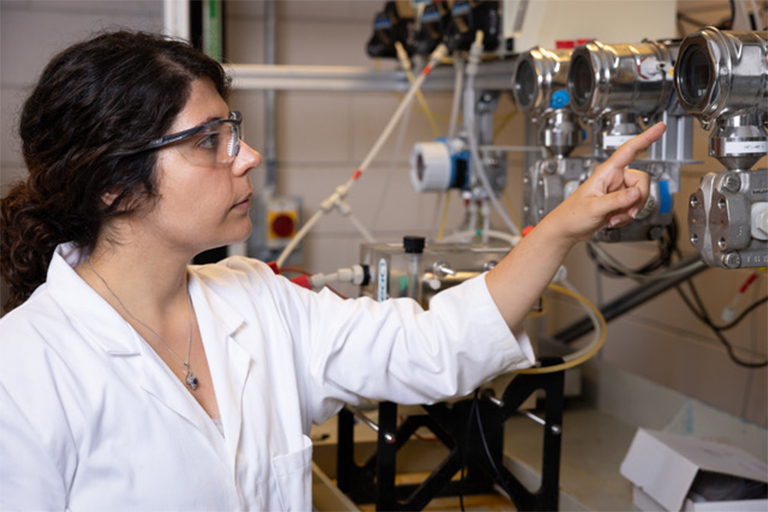
DOC offers some advantage regarding the measurement problem, as the stream of CO2 taken from the sea can be calculated, says Daan Reijnders at SeaO2, a Dutch company that is developing a DOC pilot plant that aims to store carbon geologically or put it to use. “What’s important is that we have an understanding of how that translates — and at which time scales — to CO2 removal from the atmosphere,” he says.
Research suggests that natural mCDR ocean uptake of carbon dioxide happens over a prolonged period, up to a year or more, depending on the region, and on other factors. Consequently, MRV will depend on both advanced sensor readings and modeling.
For researcher Nicholas Ward, at Pacific Northwest National Laboratory (which is running a small OAE pilot with Ebb Carbon), accurate MRV requires development of better CO2 drawdown measurement sensors than currently available. As with other CDR methods, the creation of these measurement devices can increase operational costs considerably, and until they are created, can shake investor confidence as to actual CO2 drawdown.
“It’s really hard to measure that change well beyond the pipe” in the open sea, Ward says. “That’s the challenge. The technology wouldn’t scale if it couldn’t be verified, because no one would pay for the credit.”
Ultimately, the gigantic scale at which these systems will need to operate will make accurate MRV unfeasible, according to James Kerry, senior marine and climate scientist at OceanCare, an NGO. “Reliable MRV in the open ocean is simply not possible, owing to the complexity of ocean hydrodynamics, as well the timescales and area over which it would need to operate,” he says.
He offers this example: “When we add milk to a cup of tea or coffee, it mixes differently each time. Now, imagine trying to accurately predict the mixing of treated seawater and the resulting CO2 drawdown in the Pacific Ocean over decades.”
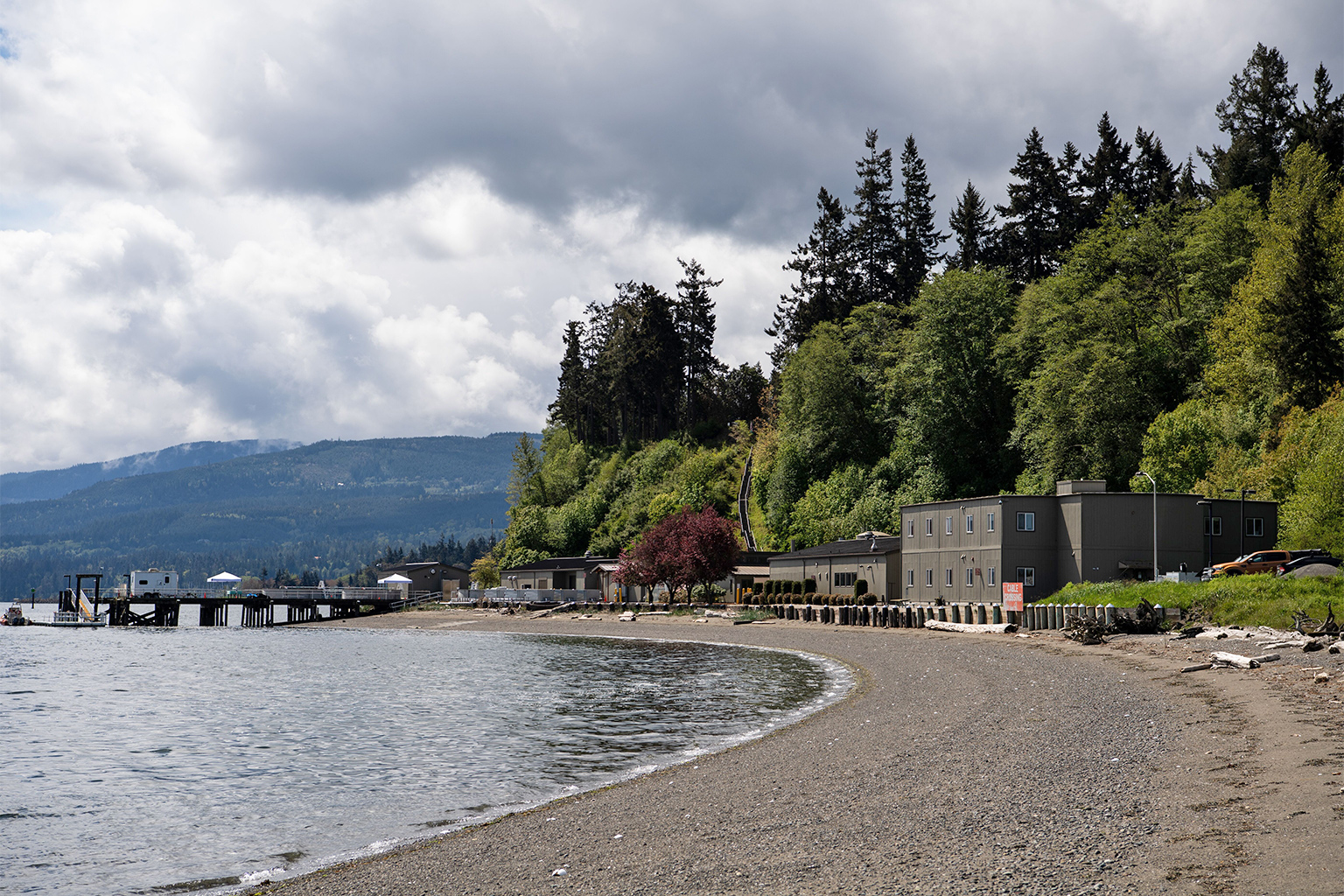

Scaling up, but at what cost?
It remains early days for mCDR, with few projects aiming above the prototype phase. That troubles some researchers when they assess the Herculean effort yet needed to scale up electrochemical plants and the potential inability to ever accurately forecast outcomes. They also wonder how large-scale electrochemical mCDR might impact the environment, including coastal ecosystems, a futuristic problem lacking in research.
“To get to the gigaton scale needed to actually make an impact at the climate level, you’re talking about processing about 1% of the ocean surface water every year, which is a huge amount of water,” Findlay notes.
Then there is the energy problem: Moving, manipulating and processing all that seawater will demand a mammoth amount of power. It can’t be created via fossil fuels, but must be delivered by renewable energy sources.
“The power that’s driving that electricity has to be renewable,” Doney says. “If you’re using fossil fuels to generate electricity and then using that for electrochemical carbon dioxide removal, you’ve … defeated the purpose.”
Potential environmental effects loom from the moment you start to take in ocean water, says Kerry, who warns that the gargantuan mCDR undertaking could suck up and destroy multiple microscopic plankton species or fish larvae. Today’s far smaller oceanside power plants equipped with water intakes used for cooling can cause similar harm to aquatic life.
At the other end of a scaled-up mCDR pipeline, there will be vast seawater discharges and the risk of impacts, including the potential for drastically increased ocean alkalinity or significantly depleted carbon dioxide in seawater, depending on the method used. These imbalances could alter ocean chemistry and harm fragile marine ecosystems via processes poorly understood at scale. Byproducts produced, including acids and chlorine, may also require careful management.
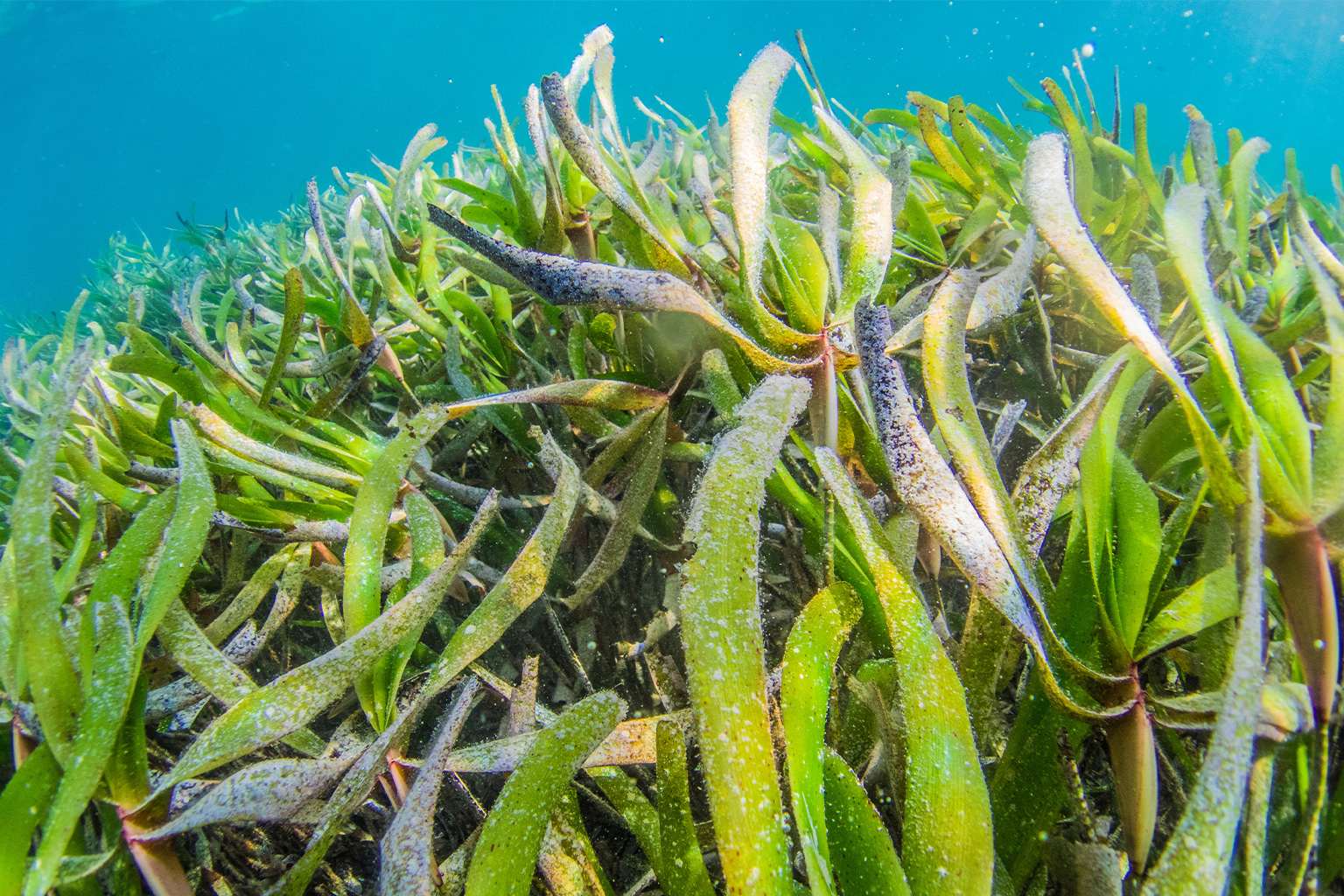
Species that need carbonate for shell formation are of particular concern, Findlay says, as are aquatic plants that rely on photosynthesis. Other experts, including Kerry, state that if operated at scale, these plants may create areas of “dead water,” impacting fisheries, local communities and livelihoods.
“I don’t think that a fear of some large impact should stop us from doing pilot demonstrations that are very unlikely to have an impact,” Ward says, but that doesn’t discount the need for careful diligence focused on “how everything from microbes to macrofauna might be impacted” once projects are scaled up.
In Ward’s view, modeling and lab tests can offer only limited data on risk. PNNL’s project, for example, has yet to deploy in open water, leaving many impact questions unanswered.
“I think we as the science community, do need to get field data to validate our models,” he says. “We have very good models for coastal systems, and we can simulate what would happen if we did this alkalinity enhancement. But without field data, it’s difficult to actually validate those [results].”
Equatic is addressing some of these concerns by siting its project near renewable energy sources while producing clean hydrogen that can be funneled back to power its mCDR system. Creating this sort of closed-loop system helps ensure “that the local environment stays the same,” Sanders says.
Netherlands-based Sea02 is developing a pilot facility capable of storing 250 tons of CO2 per year and which includes close environmental monitoring. “The point of this pilot is to verify the effectiveness of the technology, but also to further study environmental response and create learnings if we need to scale up in the future,” Reijnders says.
“Research [on impacts] needs to be done and needs to be communicated, because it only makes sense to do mCDR if it’s safe and verifiable,” he adds.
PNNL, in collaboration with Ebb Carbon, is assessing the possibility of powering their project with marine renewable energy, using tidal or wave energy, themselves nascent industries. The firms are also investigating ways to deal with the large amounts of acid that will be produced as a by-product of their electrochemical process.
Currently that project is providing this acid to a cement manufacturer. But if this mCDR system scales up, the hydrochloric acid market would be flooded, requiring the acid be disposed of by another method. One answer: Couple mCDR with algae cultivation. That way, acid by-products could be fed to the algae, which would neutralize it while allowing the algae to grow faster and to absorb more CO2.
“We’re already changing the ocean. We’re acidifying [it via climate change and] we’re looking for a solution,” Ward says. But regarding climate and geoengineering impacts, “You’re sort of damned if you do and damned if you don’t.”
He adds, “I think it’s important to frame mCDR not as a solution that will let us continue to burn fossil fuels. … We’re going to need to reduce emissions and have solutions like mCDR to reach a net neutral or net negative world.”

Avoiding unintended consequences
In 2023, a group of international research and conservation organizations proposed precautionary principles for mCDR geoengineering research, with electrochemical methods among them. They emphasized the need for community engagement, transparency, verification of carbon removal as well as rigorous standardized monitoring, reporting and verification.
Other groups and experts have emphasized the need for a code of conduct and international rules and guidelines to act as guardrails. Electrochemical plants will need to be subject to local, national or regional legislation, they say.
Some analysts, including Kerry, emphasize that international rules and guidelines should be put in place now, given the risk for widespread ocean impacts by electrochemical CDR and other marine geoengineering; “I think it’s concerning if we have a piecemeal regulatory framework dealing with these technologies,” he says. One ocean geoengineering technique, ocean iron fertilization, is already subject to some restrictions under the London Protocol, while others, including ocean alkalinity enhancement, are under review
Findlay agrees that governance needs to be “much more stringently put in place,” noting, “What we don’t understand, and I think where the risks lie, is in how we scale this up. We don’t fully understand or appreciate how to do that in the best ways without having unintended consequences on marine organisms.”
“What we’re doing is trying to show, and learn and prove whether it’s safe and effective,” all at once, says SeaO2’s Reijnders. “All projects do need to comply with permitting requirements. … In the Netherlands and the rest of the EU, [such projects] are all based on the European Water Framework Directive.”
As mCDR momentum builds and electrochemical plants of increasing size come online, some now open questions may be answered one way or the other. Those findings could ultimately determine at what scale these projects operate, or if they sputter to a halt.
But for experts such as Kerry, far more scrutiny is required now with the precautionary principle in full operation.
“The research should be focused primarily on modeling and on lab trials, before they jump to field trials, and certainly before they start to commercialize these projects and sell carbon credits,” he says. “The state we’re in with the industry right now is that the rush to commercialization is problematic.”
Banner image: The world’s oceans naturally absorb vast amounts of atmospheric carbon dioxide. Companies and scientists are investigating electrochemical approaches to coax the oceans to store more carbon, or strip it from seawater for storage or use on land. Image by Tiluria via Pixabay (Public domain).
Re-carbonizing the sea: Scientists to start testing a big ocean carbon idea
Citations
Aleta, P., Refaie, A., Afshari, M., Hassan, A., & Rahimi, M. (2023). Direct ocean capture: The emergence of electrochemical processes for oceanic carbon removal. Energy & Environmental Science, 16(11), 4944-4967. doi:10.1039/d3ee01471a
Sharifian, R., Boer, L., Wagterveld, R., & Vermaas, D. (2022). Oceanic carbon capture through electrochemically induced in situ carbonate mineralization using bipolar membrane. Chemical Engineering Journal, 438, 135326. doi:10.1016/j.cej.2022.135326
Doney, S. C., Wolfe, W. H., McKee, D. C., & Fuhrman, J. G. (2024). The science, engineering, and validation of marine carbon dioxide removal and storage. Annual Review of Marine Science. doi:10.1146/annurev-marine-040523-014702
A research strategy for ocean-based carbon dioxide removal and sequestration. (2022). doi:10.17226/26278
Karunarathne, S., Andrenacci, S., Carranza-Abaid, A., Jayarathna, C., Maelum, M., Skagestad, R., & Aksel Haugen, H. (2025). Review on CO2 removal from ocean with an emphasis on direct ocean capture (DOC) technologies. Separation and Purification Technology, 353, 128598. doi:10.1016/j.seppur.2024.128598
La Plante, E. C., Simonetti, D. A., Wang, J., Al-Turki, A., Chen, X., Jassby, D., & Sant, G. N. (2021). Saline water-based mineralization pathway for gigatonne-scale CO<sub>2</sub>Management. ACS Sustainable Chemistry & Engineering, 9(3), 1073-1089. doi:10.1021/acssuschemeng.0c08561
Jersild, A., & Landschützer, P. (2023). A spatially explicit uncertainty analysis of the air-sea CO2 flux from observations. doi:10.5194/egusphere-egu23-4184
Manley, J. E. (2024, April). Technology Requirements for Monitoring Reporting and Verification of Ocean Carbon Dioxide Removal. Paper presented at IEEE, Singapore. doi:10.1109/OCEANS51537.2024.10682413
Yafiee, O. A., Mumtaz, F., Kumari, P., Karanikolos, G. N., Decarlis, A., & Dumée, L. F. (2024). Direct air capture (DAC) vs. direct ocean capture (DOC)–A perspective on scale-up demonstrations and environmental relevance to sustain decarbonization. Chemical Engineering Journal, 497, 154421. doi:10.1016/j.cej.2024.154421
Ferderer, A., Chase, Z., Kennedy, F., Schulz, K. G., & Bach, L. T. (2022). Assessing the influence of ocean alkalinity enhancement on a coastal phytoplankton community. European Geosciences Union. doi:10.5194/bg-2022-17
Hibbeln, C. F., Marsh, P., Myers, C. R., Valdez, P. J., Edmundson, S. J., & Subban, C. V. (2024). Maximizing Marine Carbon Removal by Coupling Electrochemical and Biological Methods. Environmental Science & Technology Letters, 11, 5. doi:10.1021/acs.estlett.4c00107
FEEDBACK: Use this form to send a message to the author of this post. If you want to post a public comment, you can do that at the bottom of the page.