- Insects have been incredibly successful in developing ways of flying, with an ultra-fast flapping mode that scientists thought had evolved multiple times over history.
- Now, researchers have genetically traced that mode back to a common ancestor, a major breakthrough in understanding insect flight evolution.
- To confirm their findings, the researchers built a moth-sized robot that mimicked the various ways insects take to the sky.
Swatting a mosquito is a frustrating task as it buzzes away, its wings carrying it faster than seems physically possible. Amidst the vast animal kingdom, insects have evolved and maintained flight across the greatest number of species, making them the most successful—and in some ways, the most mysterious.
Biologists know that insects evolved two modes of flight. In one mode, their wing flaps are synchronized with neural signals from their brain. In the other, a single muscle twitch activates ultra-fast wingbeats that race past what the brain can do, such as the mosquito’s ability to flap 800 times in one second. Because these two modes appear across different insect groups, entomologists believed the skill to produce rapid wingbeats evolved independently many times.
But in a recent study in Nature, scientists discovered that a single ancestor to today’s flying insects diverged from the synchronized mode of flying and evolved those ultra-fast flight twitches more than 400 million years ago.
“That fundamentally changes the way we look at insect flight muscle in evolutionary history,” said the study’s co-lead author, Brett Aiello, a biologist and physiologist at Seton Hill University in Greensburg, Pennsylvania, in the U.S. Aiello and his colleagues traced this evolutionary path by using genetic data to construct the likely history of insect flight evolution.

The ability to flap wings much faster than signals from the brain can trigger, called asynchronous flight, exists in four insect groups: beetles, bees, true bugs, and flies. At small sizes, it allows insects to overcome the fundamental speed limit of how fast their brain can fire a signal to flap their wings. It leads to incredibly quick flight—and split-second aerial maneuvers—among those insect orders.
But many insects still fly by activating each wingbeat with a nerve signal, called synchronous flight. After the team’s genetic detective work revealed a common ancestor among those groups, they determined that some insects had returned to synchronous flight. Those insects, referred to as secondarily synchronous, would have muscles preserving evidence that they’d made the switch. Indeed, the insect they studied, the tobacco hawk moth (Manduca sexta), has those clues.
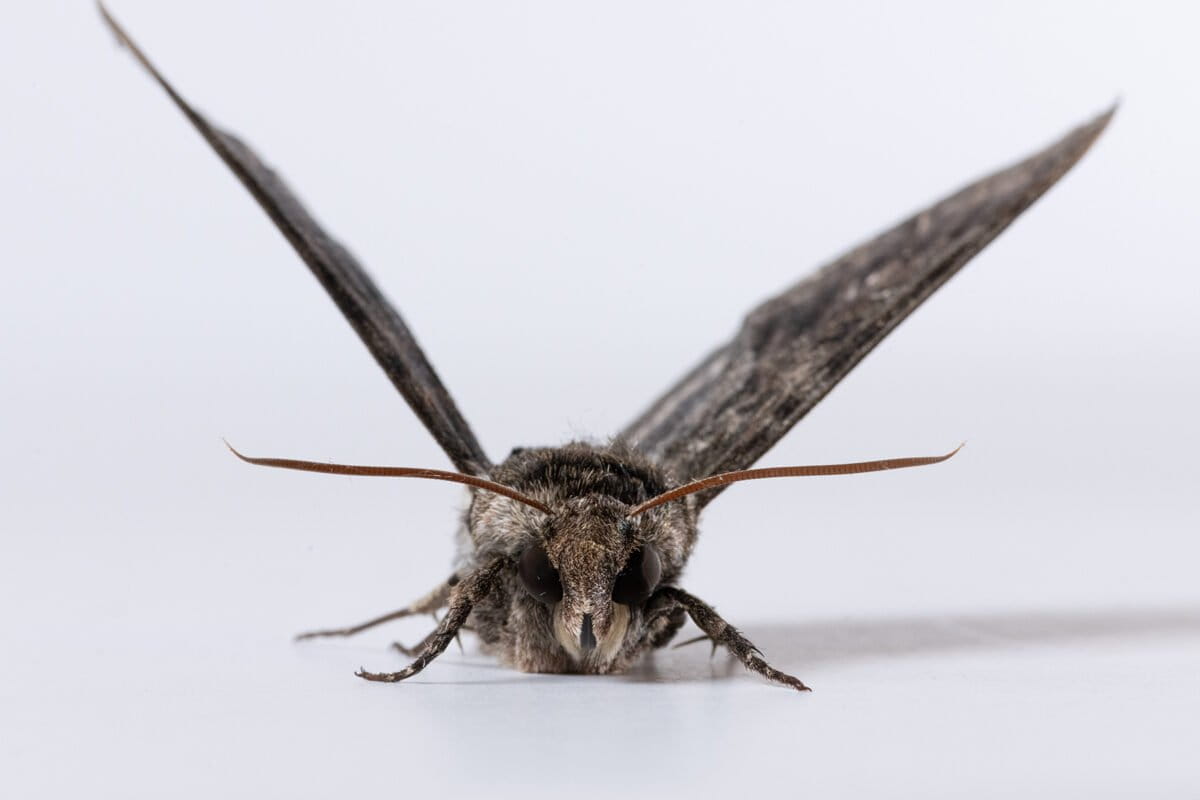
Simply watching a moth flutter wouldn’t be enough. The team wanted to know whether it was possible for the tobacco hawk moth to switch between the flight strategies. The team created a mathematical equation that combined the two kinds of flight, which made it possible to look at flight patterns that could arise for an insect with both synchronous and asynchronous skills.
To test that equation, they would need to engineer a real-life model. Co-lead author James Lynch, a Ph.D. candidate at the University of California, San Diego, and his laboratory colleagues built an insect-sized robot that could flap asynchronously—the first of its kind. The researchers made the robot with materials similar to the membranes of insect wings.
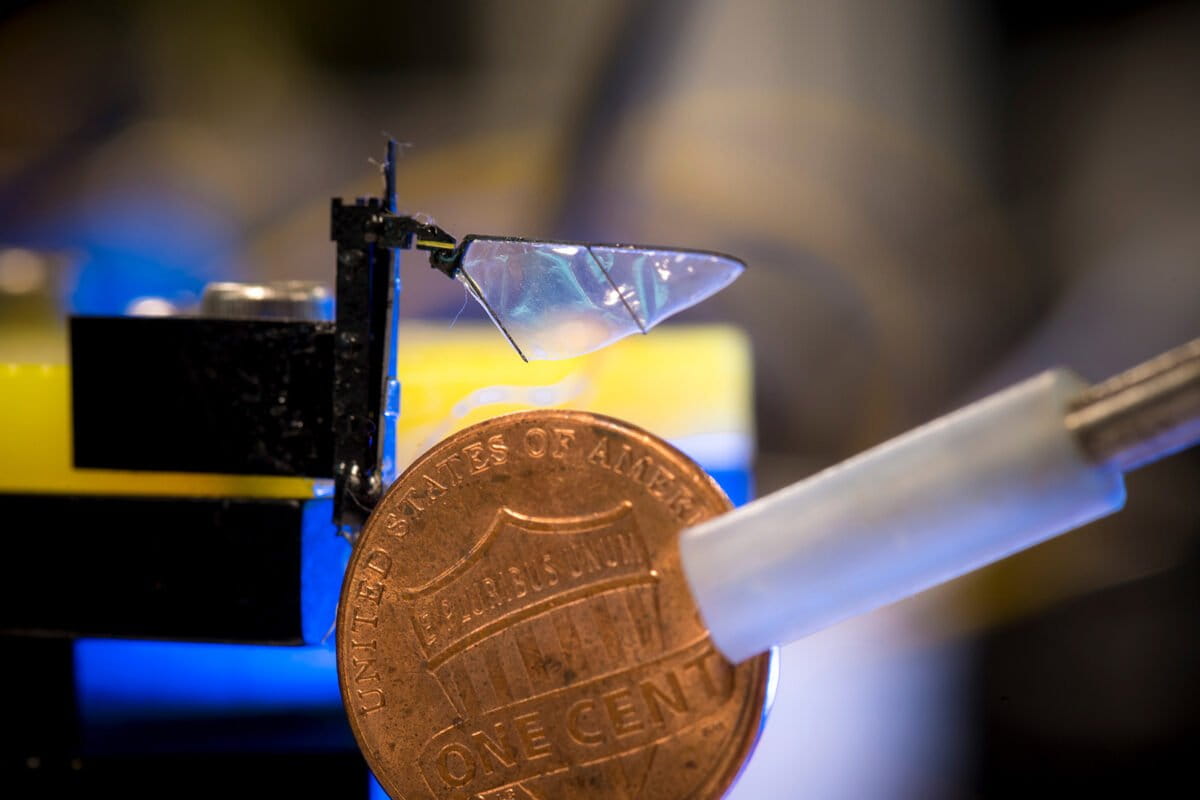

As the team changed its inputs according to their equation, the robot swapped between synchronous and asynchronous flight—and everything in between. These transitions could mimic the evolutionary bridge that allowed insects to go back and forth in their flight patterns over time, they believe.
Biophysicist John Tuthill of the University of Washington in Seattle praises the team for combining insights from evolution, muscle physiology, neurobiology, and robotics into a single study. “Bridging all those things is quite spectacular,” said Tuthill, who studies the nervous systems of fruit flies and was not involved in the research.
There is something the robot can’t do: actually fly. Though the wing portion is small, the gear powering it is far too bulky for it to soar to great heights. Still, the scientists hope their work will lead to more bio-inspired robotics in the future.
“Insects have some of the greatest sources of biological, physical, and engineering insight trapped in their little bodies, waiting to be unlocked,” said senior author Simon Sponberg, a biophysicist at the Georgia Institute of Technology in Atlanta. “If we hadn’t appreciated the diversity of insects out there, we wouldn’t have made this new technological innovation.”
Citation:
Gau, J., Lynch, J., Aiello, B., Wold, E., Gravish, N., and Sponberg, S. Bridging two insect flight modes in evolution, physiology and robophysics. Nature 622, 767–774 (2023). https://doi.org/10.1038/s41586-023-06606-3
Eli Ramos (@mxeliramos) is a graduate student in the Science Communication M.S. Program at the University of California, Santa Cruz. Other Mongabay stories produced by UCSC students can be found here.
View more recent research on insects here: